Pacific Wind and Current Changes Bring Warm, Wild Weather
If you want to understand how interconnected our planet is—how patterns and events in one place can affect life half a world away—study El Niño.
Episodic shifts in winds and water currents across the equatorial Pacific can cause floods in the South American desert while stalling and drying up the monsoon in Indonesia and India. Atmospheric circulation patterns that promote hurricanes and typhoons in the Pacific can also knock them down over the Atlantic. Fish populations in one part of the ocean might crash, while others thrive and spread well beyond their usual territory.
During an El Niño event, the surface waters in tropical waters of the central and eastern Pacific Ocean become significantly warmer than usual. That change is intimately tied to the atmosphere and to the winds blowing over the vast Pacific. Easterly trade winds (which blow from the Americas toward Asia) falter and can even turn around into westerlies. This allows great masses of warm water to slosh from the western Pacific toward the Americas. It also reduces the upwelling of cooler, nutrient-rich waters from the deep—shutting down or reversing ocean currents around the equator and along the west coast of South and Central America.
The circulation of the air above the tropical Pacific Ocean responds to this tremendous redistribution of ocean heat. The typically strong high-pressure systems of the eastern Pacific weaken, thus changing the balance of atmospheric pressure across the eastern, central, and western Pacific. While easterly winds tend to be dry and steady, Pacific westerlies tend to come in bursts of warmer, moister air.
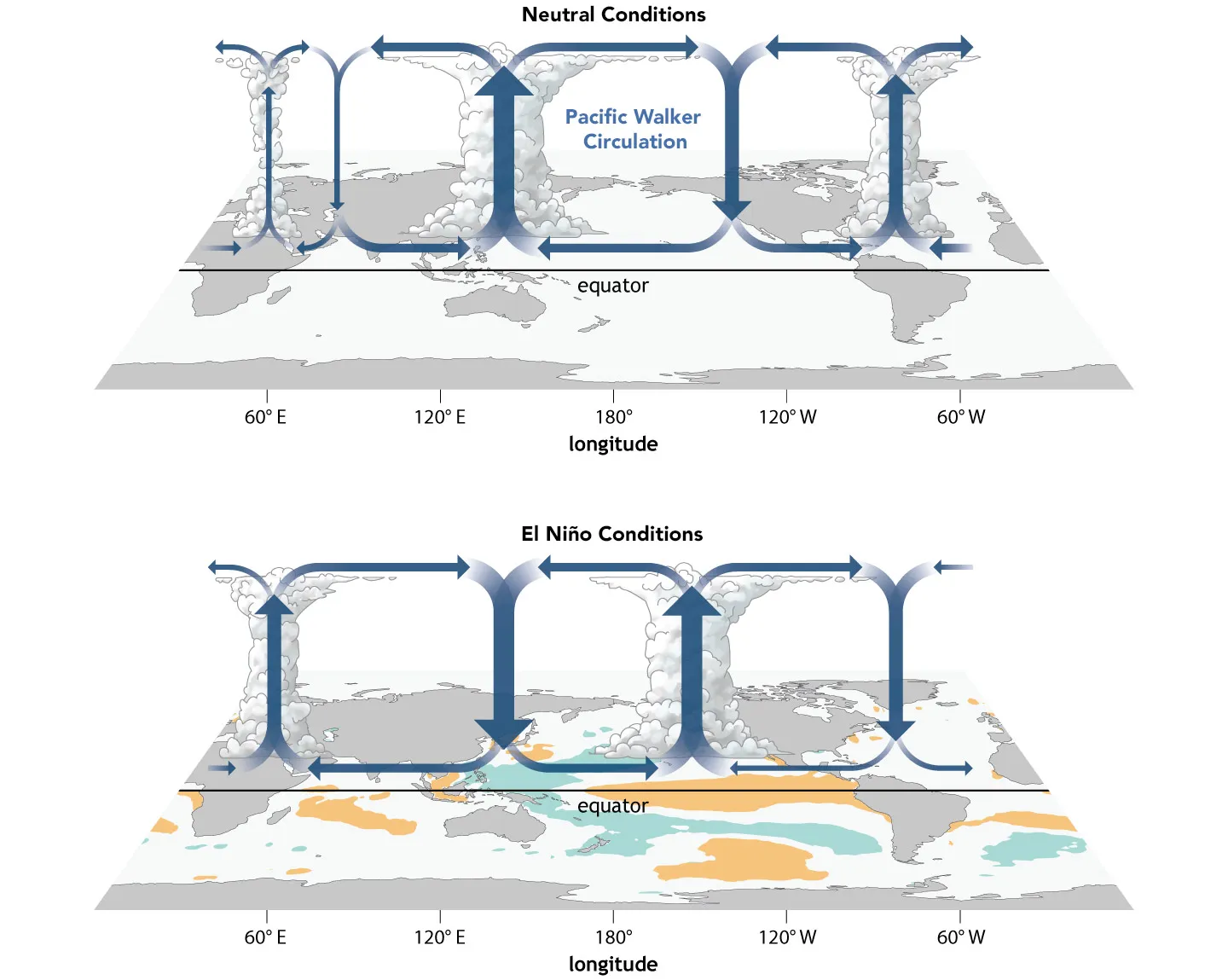
Because of the vastness of the Pacific basin—covering one-third of the planet—these wind and humidity changes get transmitted around the world, disrupting circulation patterns such as jet streams (strong upper-level winds). We know these large-scale shifts in Pacific winds and waters initiate El Niño. What we don't know is what triggers the shift. This remains a scientific mystery.
What is not a mystery is that El Niño is one of the most important weather-producing phenomena on Earth, a "master weather-maker," as author Madeleine Nash once called it. The changing ocean conditions disrupt weather patterns and marine fisheries along the west coasts of the Americas. Dry regions of Peru, Chile, Mexico, and the southwestern United States are often deluged with rain and snow, and barren deserts have been known to explode in flowers. Meanwhile, wetter regions of the Brazilian Amazon and the northeastern United States often plunge into months-long droughts.
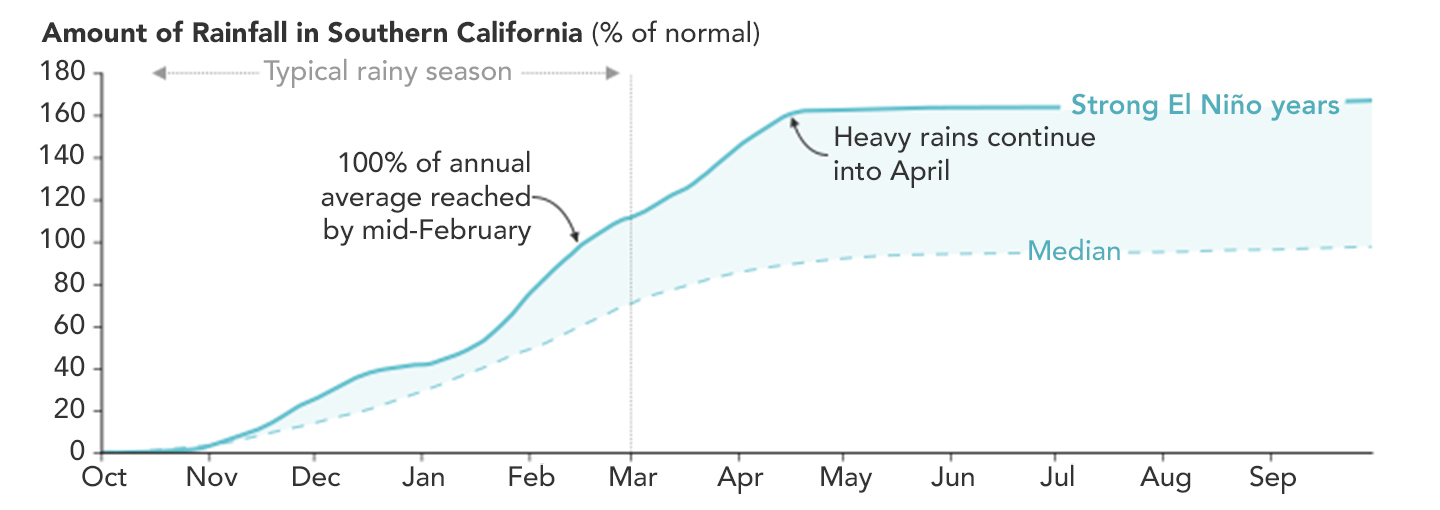
El Niño events occur roughly every two to seven years, as the warm cycle alternates irregularly with its sibling La Niña—a cooling pattern in the eastern Pacific—and with neutral conditions. El Niño typically peaks between November and January, though the buildup can be spotted months in advance and its effects can take months to propagate around the world.
Though El Niño is not caused by climate change, it often produces some of the hottest years on record because of the vast amount of heat that rises from Pacific waters into the overlying atmosphere. Major El Niño events—such as 1972–73, 1982–83, 1997–98, and 2015–16—have provoked some of the great floods, droughts, forest fires, and coral bleaching events of the past half-century.
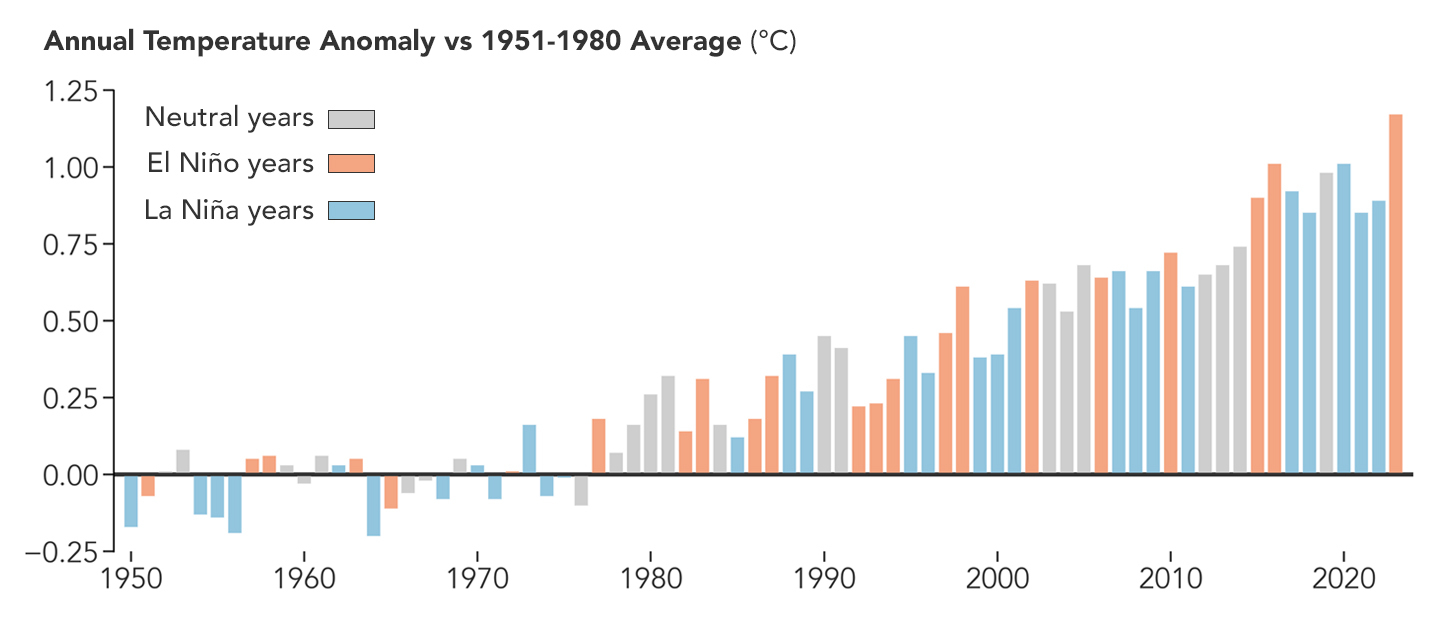
NASA, the National Oceanic and Atmospheric Administration (NOAA), and other scientific institutions track and study El Niño in many ways. From underwater floats that measure conditions in the depths of the Pacific to satellites that observe the height of the sea surface and the winds above it, scientists now have many tools to dissect this l'enfant terrible of weather. The following data visualizations show most of the key ways that we observe El Niño before, during, and after its visits.
Underwater Temperatures and Water Masses
The ocean is not uniform. Temperatures, salinity, and other characteristics vary in three dimensions, from north to south, east to west, and from the surface to the depths. With their own forms of underwater weather, the seas have fronts and circulation patterns that move heat and nutrients around ocean basins. Changes near the surface often start with changes in the depths.
The tropical Pacific receives more sunlight than any other region on Earth, and much of this energy is stored in the ocean as heat. Under neutral, normal conditions, the waters off southeast Asia and Australia are warmer and sea level stands higher than in the eastern Pacific; this warm water is pushed west and held there by easterly trade winds.
But as an El Niño pattern develops and trade winds weaken, gravity causes the warm water to move east. This mass, referred to as the "western Pacific warm pool," extends down to about 200 meters in depth, a phenomenon that can be observed by moored or floating instruments in the ocean: satellite-tracked drifting buoys, moorings, gliders, and Argo floats that cycle from the ocean surface to great depths. These in situ instruments (around 4,000 of them) record temperatures and other traits in the top 300 meters (1,000 feet) of the global ocean.
The visualization above shows a cross-section of the Pacific Ocean from January 2015 through December 2016. It shows temperature anomalies; that is, how much the temperatures at the surface and in the depths ranged above or below the long-term averages. Note the warm water in the depths starting to move from west to east after March 2015 and peaking near the end of 2015. (The western Pacific grows cooler than normal.) By March 2016, cooler water begins moving east, sparking a mild La Niña in the eastern Pacific late in 2016, while the western Pacific begins to warm again.
Sea Surface Temperatures
For hundreds of years, the temperature near the water surface has been measured by instruments on ships, moorings, and more recently, drifters. Since the late 1970s, satellites have provided a global view of ocean surface temperatures, filling in the gaps between those singular points where floating measurements can be made.
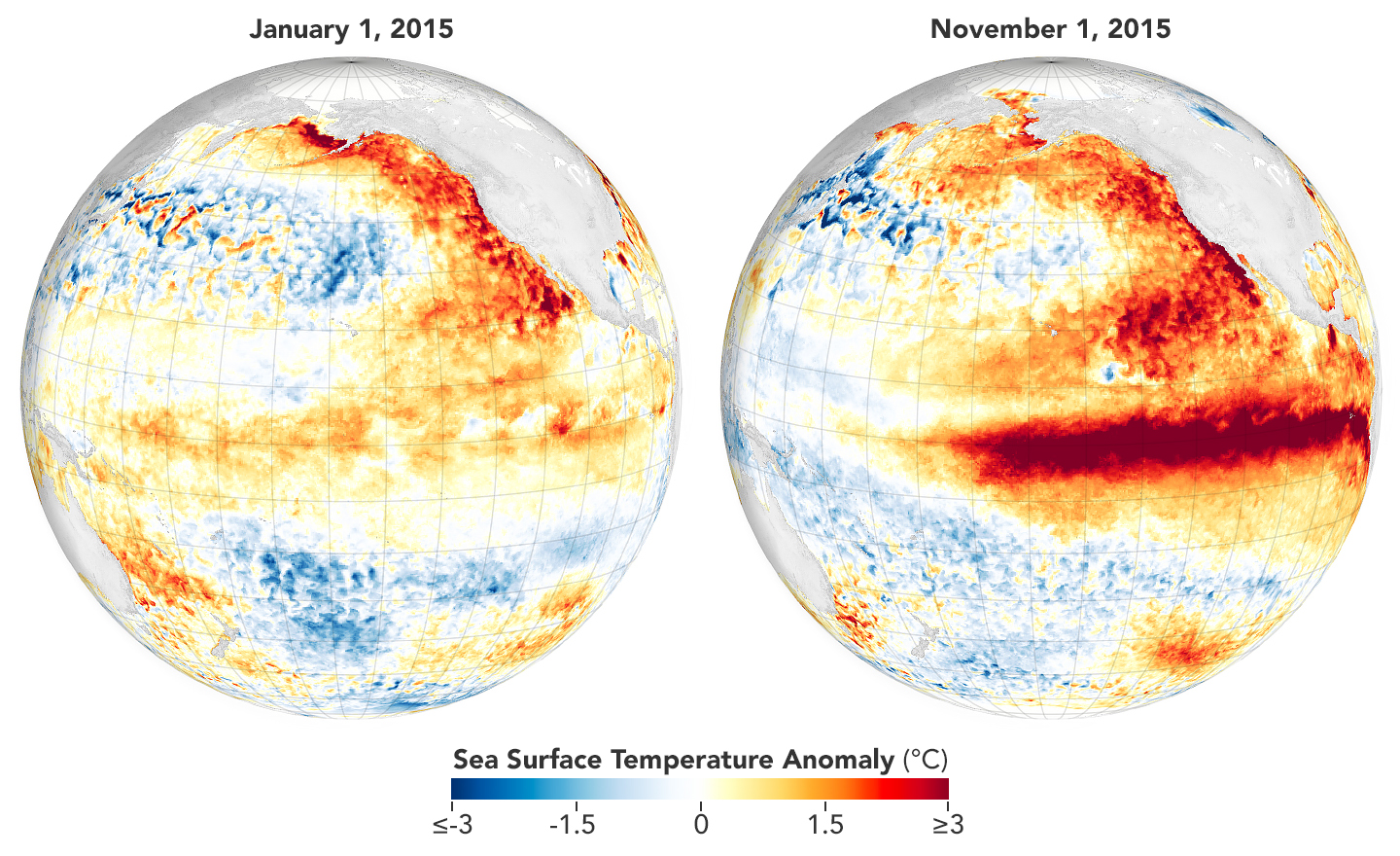
Sea surface temperatures are measured from space by radiometers, which detect the electromagnetic energy (mostly light and heat) emitted by objects and surfaces on Earth. In the case of the oceans, satellite radiometers—such as the Advanced Very High Resolution Radiometer (AVHRR) on NOAA weather satellites and the Moderate Resolution Imaging Spectroradiometer (MODIS) on NASA's Terra and Aqua satellites—detect the strength of infrared and microwave emissions from the top few millimeters of the water.
The maps above show sea surface temperature anomalies in the Pacific from the winter and fall of 2015. The maps do not depict absolute temperatures; instead, they show how much above (red) or below (blue) the surface water temperatures were compared to a long-term (30-year) average. The maps were built using data from the Multiscale Ultrahigh Resolution Sea Surface Temperature (MUR SST) project, an effort by NASA's Jet Propulsion Laboratory that blends measurements of sea surface temperatures from multiple NASA, NOAA, and international satellites, as well as ship and buoy observations.
When deciding whether the Pacific is in an El Niño state, the climatologists at NOAA examine sea surface temperatures in the east-central tropical Pacific—referred to as the Niño 3.4 region (between 120° and 170° West). An El Niño is declared when the average temperature stays more than 0.5 degrees Celsius above the long-term average for five consecutive months. In 2023–2024, sea surface temperatures rose about 2.0 degrees Celsius (3.6 degrees Fahrenheit) above average. During the much stronger El Niño events of 1997–98 and 2015–16, sea surface temperatures rose more than 2.5 degrees Celsius (4.5 degrees Fahrenheit) above average.
Sea Surface Height
Sea level is naturally higher in the western Pacific; in fact, it is normally about 40 to 50 centimeters (15–20 inches) higher near Indonesia than off of Ecuador. Some of this difference is due to tropical trade winds, which predominantly blow from east to west across the Pacific Ocean, piling up water near Asia and Oceania. Some of it is also due to the heat stored in the water, so measuring the height of the sea surface is a good proxy for measuring the heat content of the water.
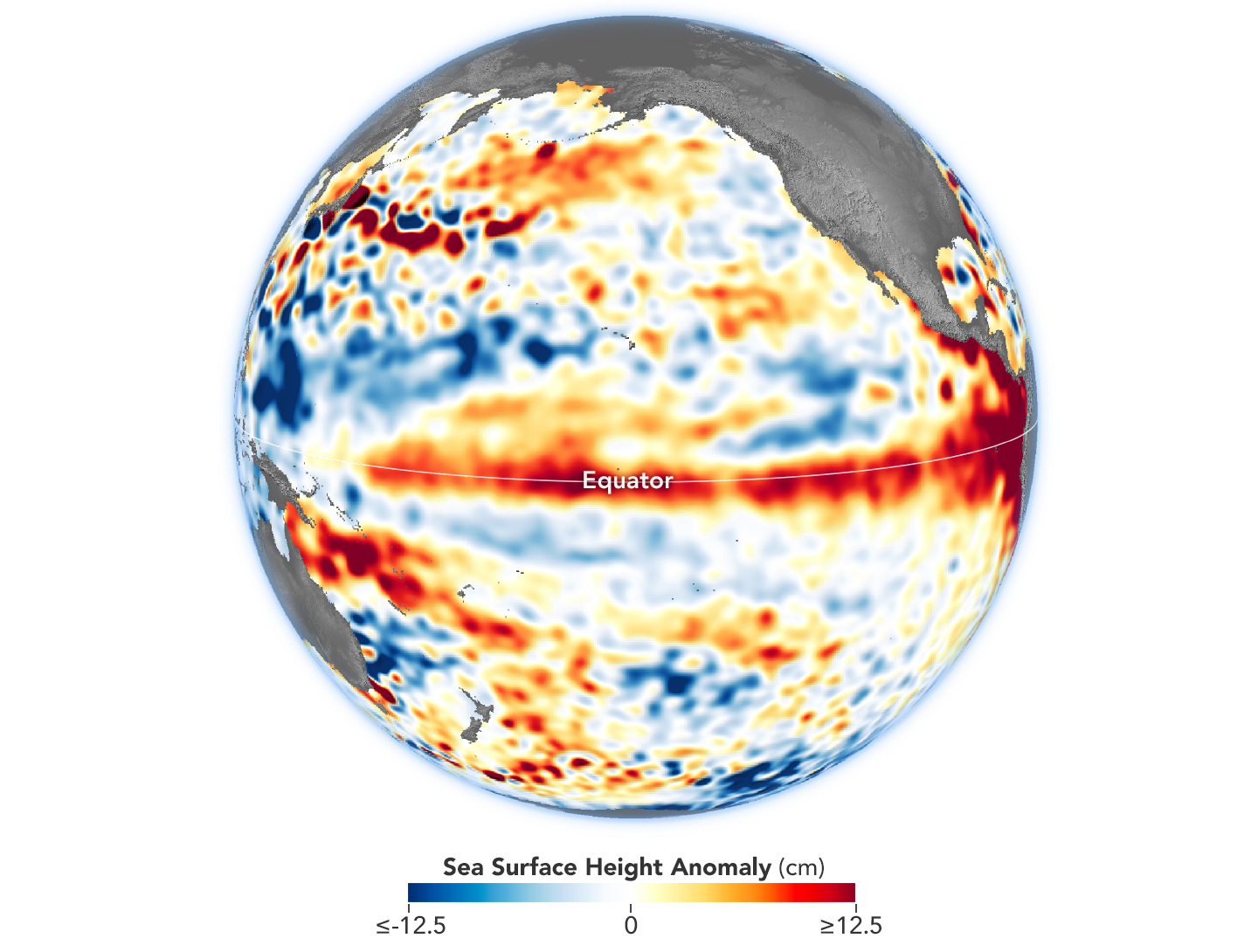
The map above shows sea surface heights in the Pacific Ocean as measured by the Sentinel-6 Michael Freilich and Sentinel-3B satellites and processed by scientists at JPL. It shows sea surface height anomalies, or how much the water stood above or below its normal sea level. Shades of red indicate where the ocean was higher because warmer water expands to fill more volume (thermal expansion). Shades of blue show where sea level and temperatures were lower than average (water contraction). Normal sea-level conditions appear in white.
Sea level is naturally higher in the western Pacific. But when the trade winds ease and bursts of wind come out of the west, warm water from the western Pacific pulses east in vast, deep waves (Kelvin waves) that even out sea level a bit. As the warm water piles up in the east, it deepens the warm surface layer, lowering the thermocline and suppressing the natural upwelling that usually keeps waters cooler along the Pacific coasts of the Americas.
Ocean Color
As temperatures change due to El Niño, other effects ripple through the ocean. In the eastern Pacific, the surge of warm water deepens the thermocline, the thin layer that separates surface waters from deep-ocean waters. This thicker layer of warm water at the surface curtails the usual upwelling of cooler, nutrient-rich water—the water that usually supports rich fisheries in the region. This loss of nutrient supply is evident in declining concentrations of sea surface chlorophyll, the green pigment present in most phytoplankton. Changes in water properties, such as oxygen and carbon content, also affect marine life.
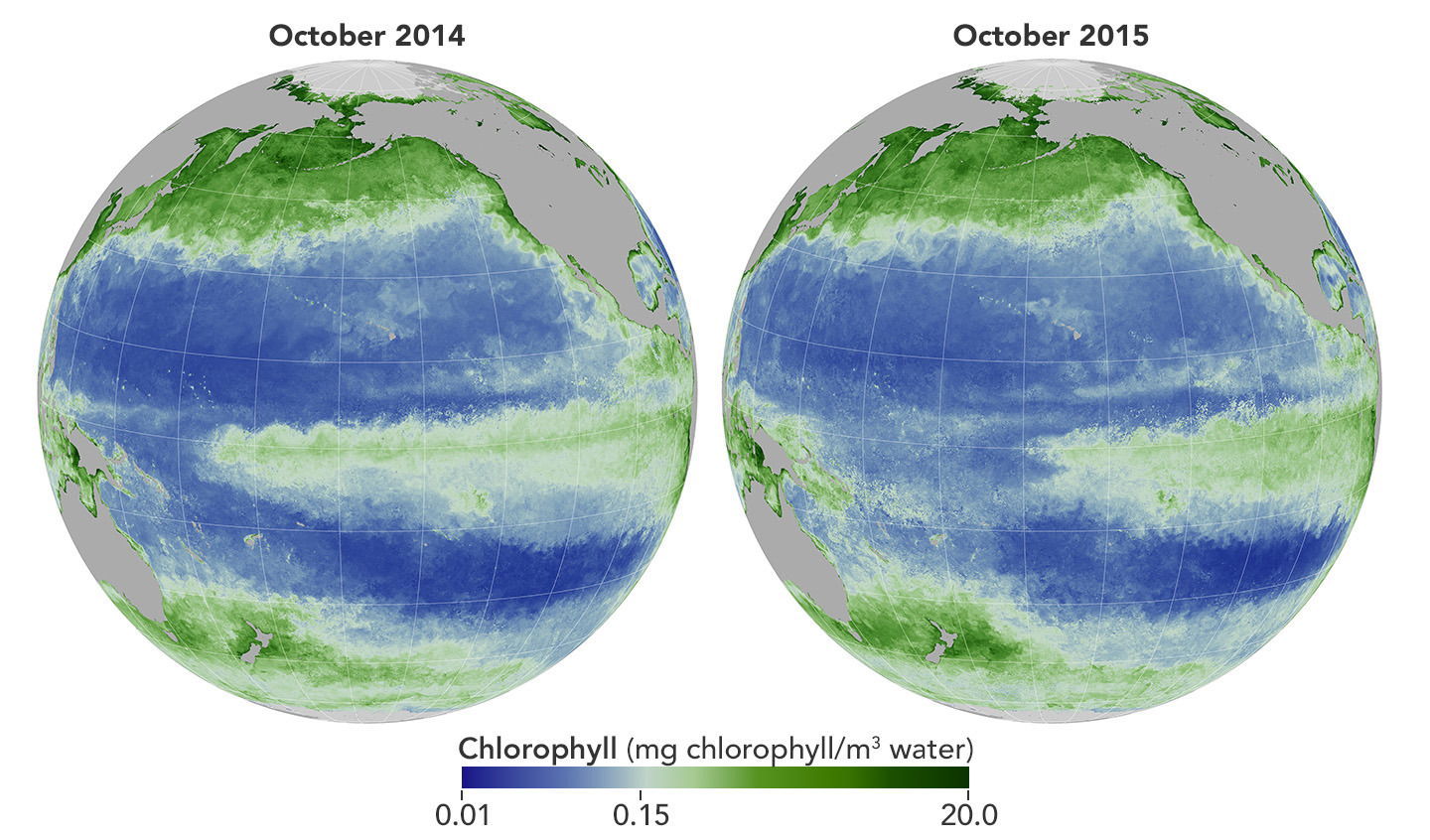
The images above compare sea surface chlorophyll in the Pacific Ocean as observed in October 2014 and 2015. Shades of green indicate more chlorophyll and blooming phytoplankton. Shades of blue indicate less chlorophyll and less phytoplankton. (For a larger view of these maps, click here.)
Historic observations have shown that with less phytoplankton available, the fish that feed on plankton—and the bigger fish that feed on the little ones—have a greatly reduced food supply. In the most extreme El Niños, the decline in fish stocks has led to famine and dramatic population declines for marine animals such as Galapagos penguins, marine iguanas, sea lions, and seals.
Surface Winds
The behavior of the winds and waters is tightly intertwined in the Pacific basin during an El Niño event. "It is like the proverbial chicken-and-egg problem," says Michael McPhaden of NOAA’s Pacific Marine Environmental Laboratory. “During an El Niño year, weakening winds along the equator lead to warming water surface temperatures that lead to further weakening of the winds.”
The maps below show the dominant direction of the winds (left) and changes in their intensity (right) near the ocean surface as observed by NASA’s RapidScat instrument. Arrows show how the primary wind direction changed from January 2015 to January 2016. The change in wind speed is represented by colors, with surface wind speeds increasing in orange areas and decreasing in purple areas.
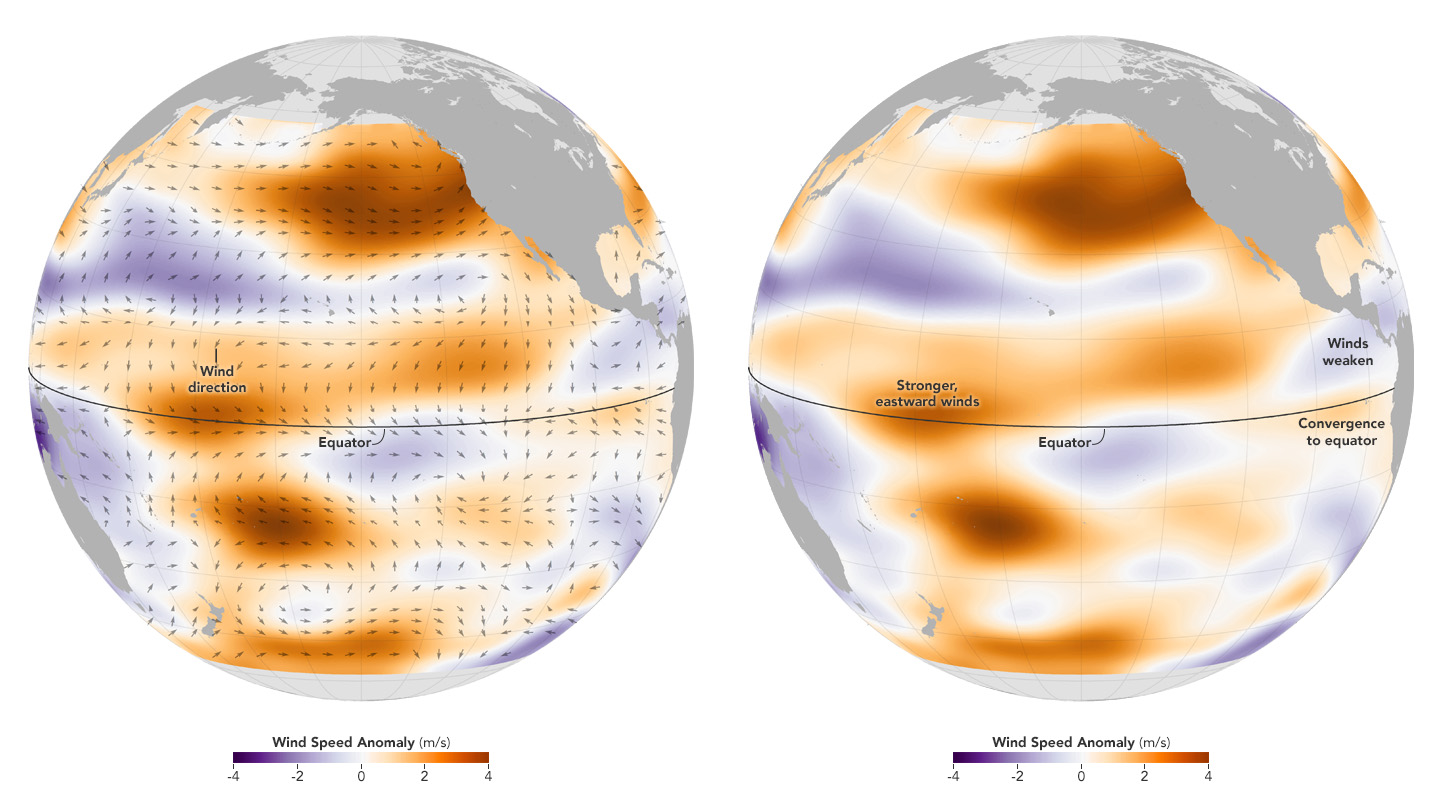
The El Niño signal is evident in the eastward-blowing winds in the tropical western and central Pacific. Winds near the equator (5° North to 5° South) blew more forcefully from west to east in the western and central Pacific; meanwhile, the easterly (east to west) trade winds weakened near the Americas. These wind shifts allowed pulses of warm water to slosh from Asia toward the Americas over the course of 2015. The signal also shows up in a convergence in the eastern Pacific; that is, the winds in the tropics (23°N to 23°S) were generally moving toward the equator. This reflects intense convection, where warm surface waters promote intense evaporation and rising air. (See the Walker circulation illustration near the top of this story.) Consequently, new air masses move toward the equator to replace the rising air.
Other changes occurred well away from the equator; scientists refer to these as teleconnections. For instance, RapidScat—an instrument mounted on the International Space Station that measured surface winds over the ocean—detected a strong clockwise-rotating (anti-cyclonic) wind anomaly in the northeastern Pacific that may have been the result of stronger-than-normal atmospheric circulation (Hadley cell). That is, air that rose above the super-heated waters of the central tropical Pacific sank back to the surface at higher latitudes with more than usual intensity.
Cloudiness and Precipitation
By changing the distribution of heat and wind across the Pacific, El Niño alters rainfall patterns for months and seasons. As the warm ocean surface warms the atmosphere above it, moisture-rich air rises and develops into rain clouds. So while the majority of precipitation tends to occur over the west Pacific warm pool in neutral years, much more develops over the central and eastern Pacific during an El Niño event.
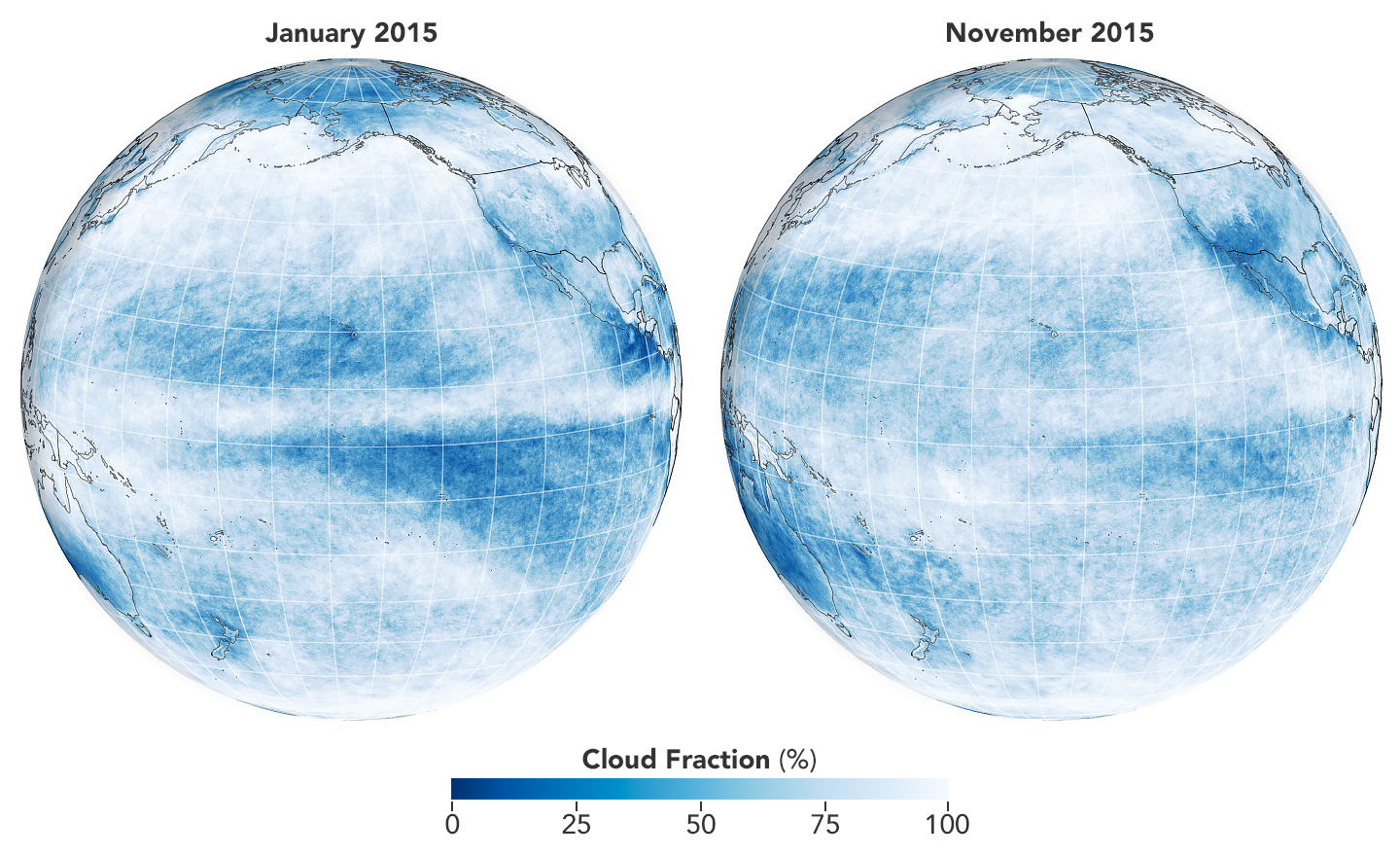
The globes show cloud fraction over the Pacific Ocean in January and November 2015, as measured by the MODIS instrument on NASA's Aqua satellite. The data show how often and how much the sky was filled with clouds over a particular region. Cloudiness is a result of moisture rising from the ocean surface into the atmosphere. During an El Niño (November image), cloud cover increases in the eastern Pacific due to the warm water releasing more moisture and heat into the atmosphere. Those clouds can lead to more rain, but they also shade the water by day and trap heat near the surface at night.
The Human History of El Niño
El Niño was identified and named long before science caught up with the phenomenon. For centuries, Peruvian fishermen reaped a bounty off the Pacific coast of South America, where north- and west-flowing currents pulled cool, nutrient-rich water from the deep. But every so often, the currents would stop or turn around; warm water from the tropics would drive the fish away and leave the nets empty. These periodic warm spells were most noticeable around December or January—around the time of Christmas and the birth of "the boy child."
Some of the first scientific descriptions of El Niño came during exchanges between the Lima Geographical Society and the International Geographic Congress in the 1890s. But the roots of El Niño stretch far back into history, long before the birth of Jesus of Nazareth or the arrival of Peruvian fishermen. The chemical signatures of warmer seas and increased rainfall have been detected in coral samples and in other paleoclimate indicators since the last Ice Age. This pattern of water and wind changes has been going on for tens of thousands of years.
Earth scientists, historians, and archaeologists have theorized that El Niño played a role in the demise or disruption of several ancient civilizations, including the Moche, the Inca, and other cultures in the Americas. But the recorded history of El Niño really starts in the 1500s, when European cultures reached the New World and met indigenous American cultures.
16th Century
Historical research has suggested that the Spanish conquest of the Incas and Peru may have been aided by El Niño conditions. When Francisco Pizarro first sailed from Panama along the west coast of South America in 1524, his progress was slowed and ultimately stopped by persistent south and southeasterly winds, which follow the pattern of the north-flowing coastal currents. In 1525–26, however, Pizarro got much farther down the coast, riding on favorable northeasterly winds, according to geographer Cesar Caviedes, author of El Niño in History.
When Pizarro returned in 1531-32, his ships made haste down the coast, pushed along again by strong northeasterlies—the kind that blow in El Niño years. Once Spanish troops moved inland, they found blooming deserts, swollen rivers, and rainfall in the usually arid regions of Peru and Ecuador. The humid air and moist land allowed the conquistadors to sustain their long march and to avoid Incan settlements on the way to establishing a foothold in the country.
18th Century
Between 1789 and 1792, the monsoon in South Asia failed multiple times, according to historical and scientific records. There is evidence that several other climate patterns—some of them affected by or coinciding with Asian monsoon patterns and El Niño—influenced storm tracks and westerly winds near Europe. According to some researchers, the combination of climate anomalies and unusual weather led to crop failures in Europe and set the stage for some of the unrest that exploded in the French Revolution of 1789.
19th Century
In the book Late Victorian Holocausts, historian Mike Davis suggests that at least three great famines in the late 19th century were connected to El Niño. Extreme weather and the collapse of monsoon circulation—patterns documented by British and Indian officials, among others—led to great droughts and a few floods in 1876–78, 1896–97, and 1899–1900. Between 30 and 60 million people perished in India, China, and Brazil, among other countries; hundreds of millions suffered through hunger and social and political strife. Though European colonialism and the spread of laissez faire capitalism played important roles in these calamities, the global reach (teleconnections) of El Niño and La Niña likely spurred the great droughts, crop failures, and malaria outbreaks.
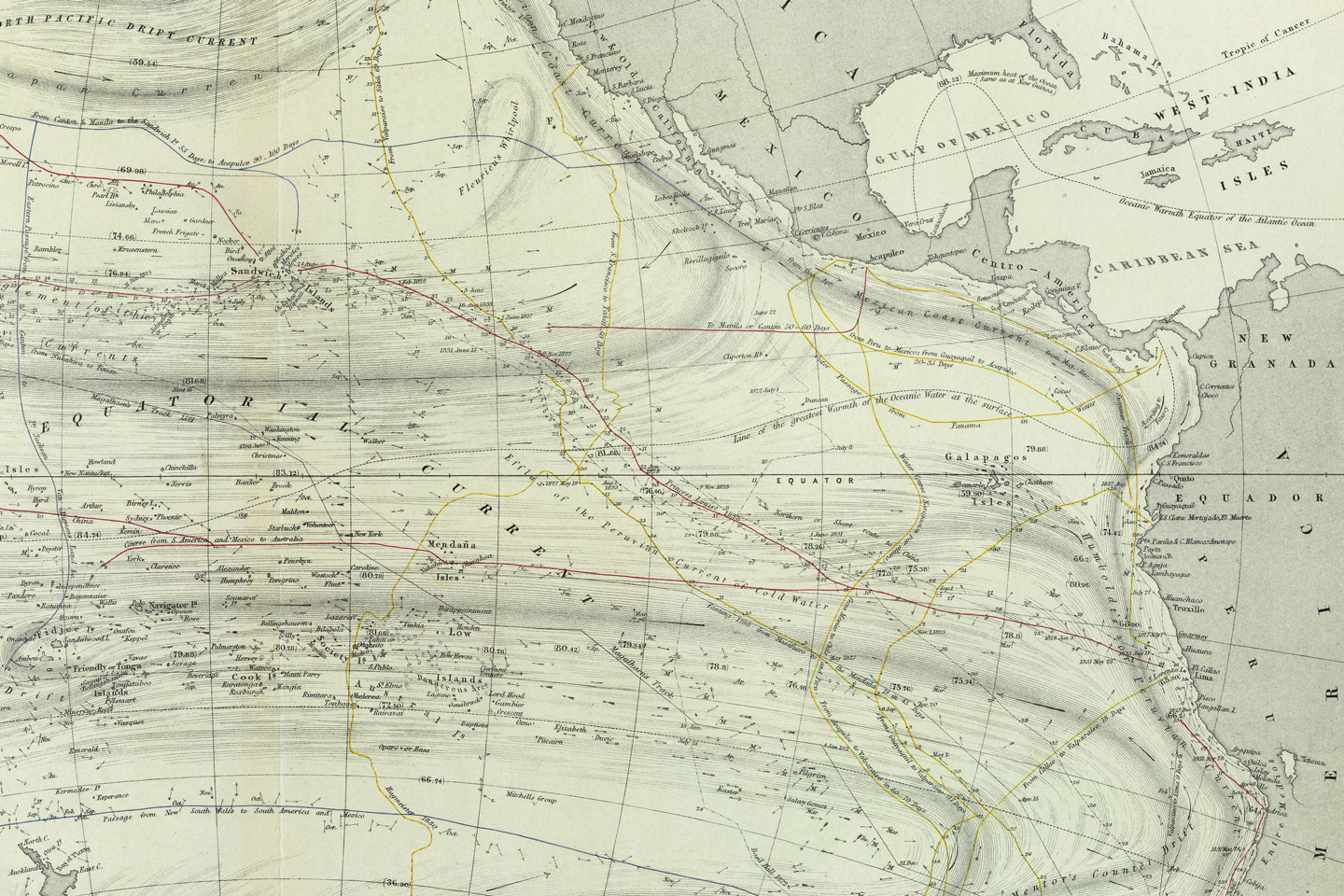
20th Century
In the 1920s, a transplanted statistician and physicist from Britain began to piece together the big picture of this global weather-maker. While working as Director of Observatories in India and studying the monsoon, Gilbert Walker noted that "when pressure is high in the Pacific Ocean it tends to be low in the Indian Ocean from Africa to Australia; these conditions are associated with low temperatures in both these areas, and rainfall varies in the opposite direction to pressure." He dubbed the alternating atmospheric weather pattern the "Southern Oscillation," noting how highs over the tropical Pacific coincided with lows over the Indian Ocean and vice versa.
It would be another four decades before Jacob Bjerknes—a Norwegian-born scientist who helped found the meteorology department at the University of California, Los Angeles—made the final connection between the alternating warm and cool patterns in Pacific waters and the atmospheric circulation described by Walker. The entire pattern came to be known as ENSO, or El Niño-Southern Oscillation, and it includes the sister phenomenon known as La Niña.
At least 27 El Niños were recorded in the 20th century, and each brought its own wrinkles that piqued the interest of scientists and sent ripples through economies. The El Niño of 1957–58, for instance, caused serious damage to the kelp forests off California. Another event in 1965–66 crashed the market for guano (fertilizer) in Peru and also spurred the use of soybeans for animal feed (instead of fish meal). In 1972–73, the anchovy population crashed, leading to the deaths of millions of sea birds and destabilizing effects on the Peruvian economy and government.
In 1982–83, the first major El Niño to get significant real-time study, sea birds on Christmas Island abandoned their young and flew out over the Pacific in a desperate search for food. Nearly 25 percent of the fur seal and sea lion populations off Peru starved to death.
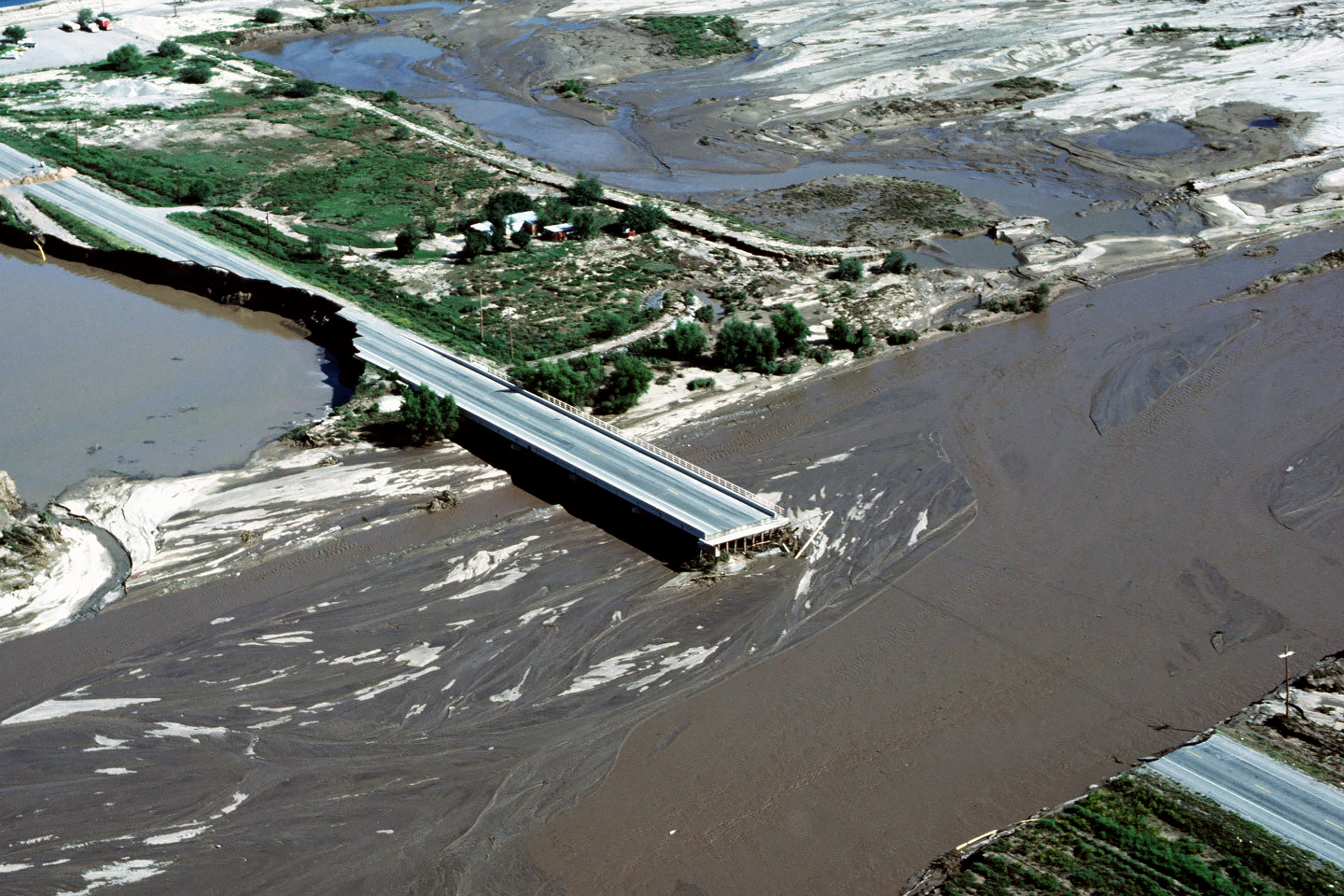
"To ask why El Niño occurs is like asking why a bell rings or a pendulum swings," atmospheric scientist George Philander wrote in a 1999 paper. "It is a natural mode of oscillation. A bell, of course, needs to be struck in order to ring." After nearly 100 years of investigation, scientists are still not sure what rings the bell; they just know that it rings.
Impacts and Teleconnections
Fires raging in Indonesia. Fisheries collapsing off Peru. Delayed monsoon rains over India. Floods and mosquito-borne disease outbreaks in South America. Epic drought and mass migrations in southern Africa. Coral die-offs in all major ocean basins. Once an El Niño is declared, it seems every extreme weather-related event in the world is blamed on this phenomenon.
El Niño is the largest natural disruption to the Earth system, with direct impacts across most of the Pacific Ocean. Indirect impacts reverberate around the globe in patterns that scientists refer to as teleconnections. Scientists are actively trying to understand how these changes in weather patterns in one area can alter the movement of air masses and winds in areas adjacent to and even far away from the source. According to the International Research Institute for Climate and Society at Columbia University, the El Niño-Southern Oscillation is responsible for as much as 50 percent of year-to-year climate variability in some regions of the world.
So which impacts are actually typical and traceable to El Niño?
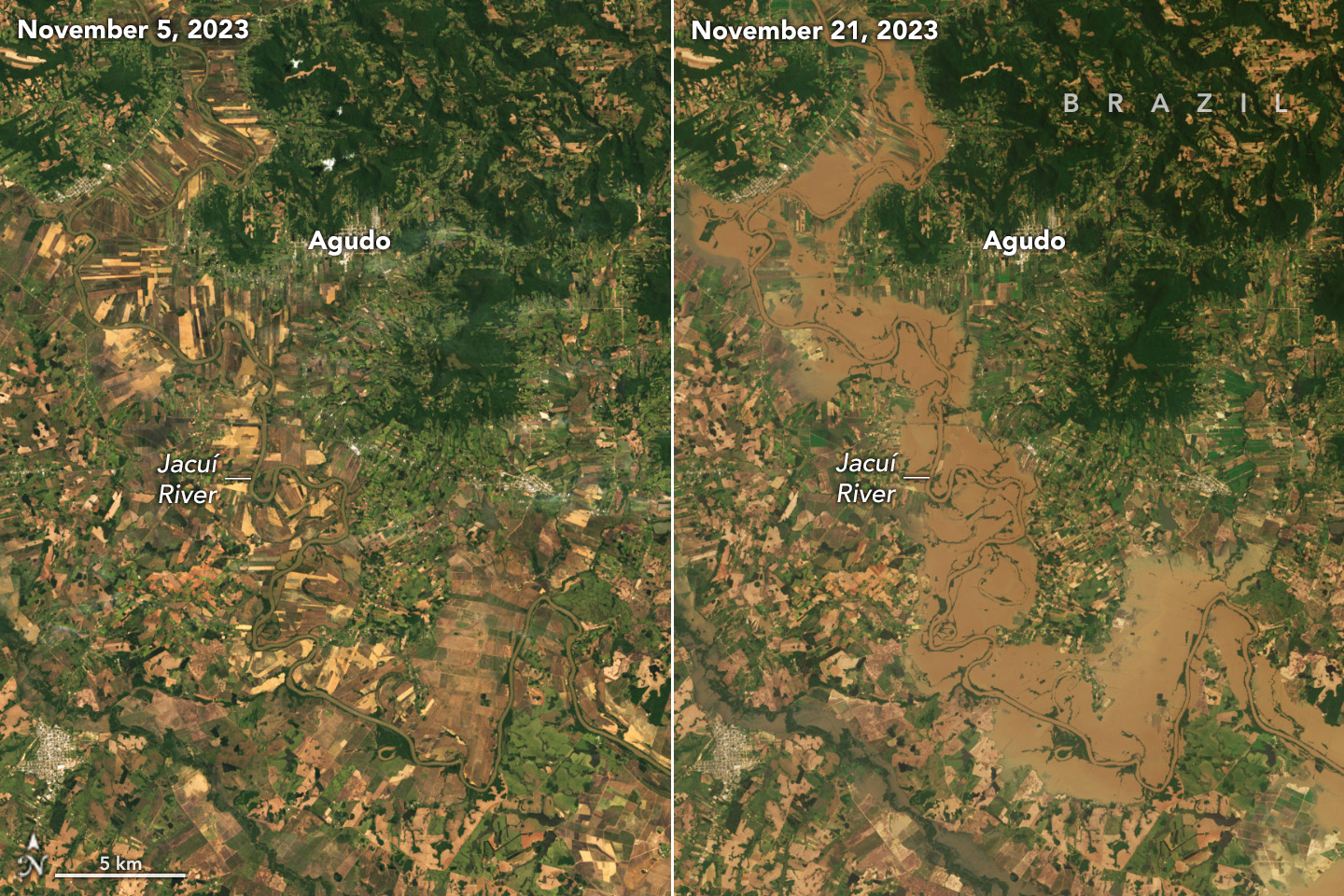
The effects are most immediate in the equatorial Pacific. The thicker, warmer surface layer of water in the eastern Pacific suppresses the upwelling of cooler, nutrient-rich water from the depths. Less nutrients mean less phytoplankton, which in turn leads to hunger all the way up the marine food web. Higher forms of marine life across the tropical Pacific—such as tuna, sea turtles, and seabirds—move to different feeding grounds when nutrient-poor surface waters shift eastward from the western Pacific.
Subtle changes in the color of the ocean—which indicate shifts in the abundance and location of the phytoplankton (visible via the pigment chlorophyll-a)—were first observed from space by the Coastal Zone Color Scanner (CZCS) in the 1970s and 80s. In fact, imagery collected by CZCS during the very strong 1982–83 El Niño showed the regional demise of marine life around the Galapagos Islands. Fifteen years later, the Sea-viewing Wide Field-of-view Sensor (SeaWiFS) compiled the first high-quality, Pacific-wide view of chlorophyll-a during the very strong 1997–98 El Niño.
In one ironic twist, rainfall increases over the eastern Pacific during an El Niño, which benefits life on the land. Even though life in the ocean is starving or moving to new feeding grounds, the plants and animals of the Galapagos and along the west coasts of North and South America usually get bountiful rain.
Although El Niño has the most direct impacts on life in the equatorial Pacific, its effects propagate north and south along the coast of the Americas, affecting marine life all around the Pacific and the Caribbean.
According to Dan Rudnick of the Scripps Institution of Oceanography, changes in oceanic and atmospheric circulation off the coast of California—primarily changes in winds—decrease the normal upwelling of cooler, deep water. Additionally, atypical ocean currents can bring tropical species to California waters, such as manta rays, red tuna crabs, and yellow-bellied sea snakes.
Warm water in the eastern and central Pacific—and the moisture and energy it gives up to the atmosphere—fuels nascent tropical storms, enabling them to develop into hurricanes. Tropical storm development is also aided by typical El Niño wind patterns, which tend to have the same horizontal wind speeds and directions both near the surface and at higher altitudes. This lack of differences in wind velocity with height (that is, a lack of "wind shear") enables storms to continue pumping heat upward and to grow stronger. By contrast, there is greater wind shear over the Atlantic Ocean and Caribbean Sea during an El Niño, which inhibits hurricane formation by dissipating the upward motion of heat.
In the equatorial Pacific, as the warm pool propagates eastward, clouds and rainfall move with it and leave the western Pacific in dry conditions that often lead to drought across Indonesia, southeast Asia, and northern Australia. The problems of drought are compounded by slash-and-burn land clearing.
For example, in Indonesia, it is common for farmers to clear-cut forests for lumber and to burn rainforests to develop the land. Normally, these fires are extinguished by the consistent rains that fall in the tropics. But when the rain dries up during a strong El Niño, those fires burn uncontrolled. Massive El Niño-fueled fires were blamed for thousands of premature deaths from air pollution in 1997–98 and contributed to as many as 100,000 deaths in 2015–16, according to a study by Harvard University scientists.
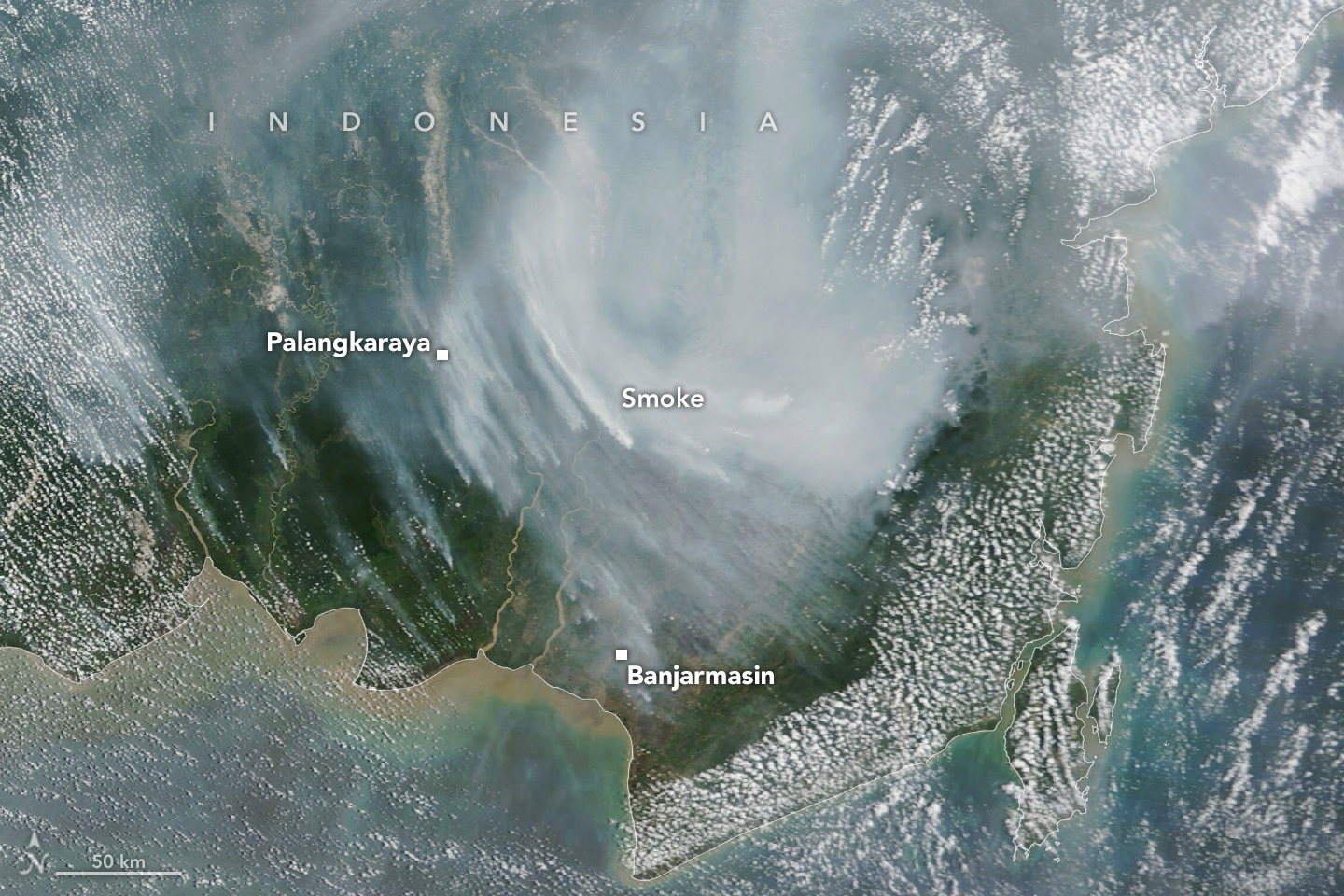
Wildfires also release extra carbon dioxide (CO2) into the air. Vegetation that is stressed by heat and drought cannot absorb as much atmospheric carbon as it normally takes up during photosynthesis. Because of this, atmospheric CO2 (as measured at the Mauna Loa observatory in Hawaii) has less of a seasonal decline during the Northern Hemisphere growing season. Thus, the rise in atmospheric CO2 is more pronounced during El Niño years.
While the impacts of an El Niño are predominately felt in the tropical Pacific, the massive reorganization of ocean heat, clouds, rainfall, and winds can affect weather patterns in other parts of the world. The atmospheric jet stream becomes faster and shifts its position, displacing the usual location of high- and low-pressure systems and altering normal storm tracks. This, in turn, modifies wet and dry areas, causing some places to experience droughts while others may get floods, landslides, and a redistribution of groundwater.
Central America typically becomes warmer and drier during El Niño years. In 1998, 2015, and 2023, reduced rainfall led to low water in the Panama Canal, causing operators to restrict some large ships from making the passage.

In South America, Brazil typically experiences unusual heat. Less rain falls in the north, while more falls from southern Brazil to Argentina. Flooding in January 2016 displaced more than 150,000 people in Uruguay, Paraguay, and Argentina and caused Paraguay’s Ministry of Public Health and Social Welfare to declare an alert for mosquito-borne diseases such as dengue, chikungunya, and Zika.
Although the impacts of every El Niño vary, more rain typically falls during the winter across the southern United States, from California to Florida. For example, in 2015-16, extreme rainfall led to landslides in Northern California and flash floods in Louisiana and Alabama.
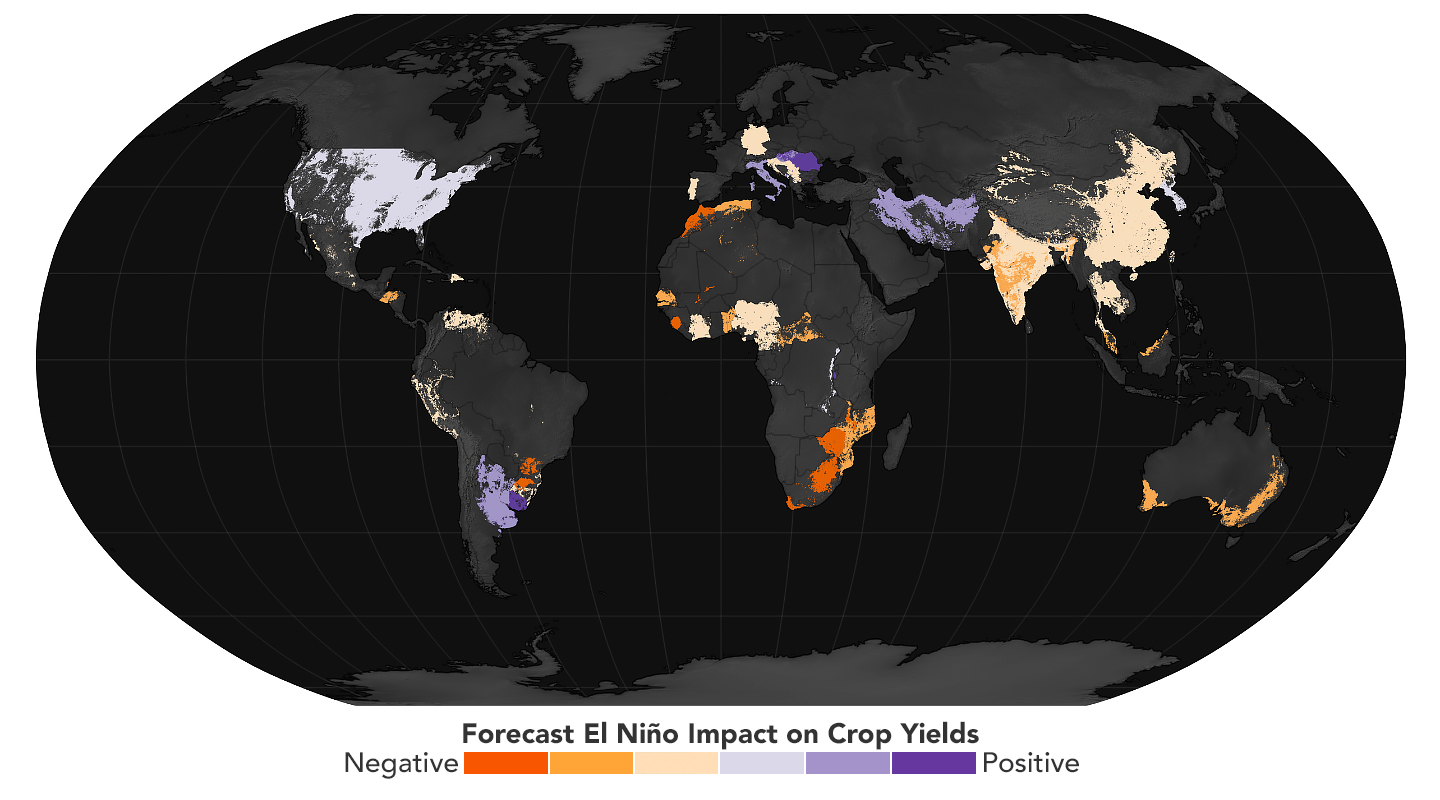
Shifting rainfall patterns across the planet can have mixed consequences for food production. During the 2023 El Niño, too much rain in some places and too little in others was projected to affect crop yields and leave 110 million people in need of food assistance, according to scientists with the Famine Early Warning Systems Network (FEWS NET). A similar scenario unfolded during the 2015-16 El Niño, when the United Nations (U.N.) Office for the Coordination of Humanitarian Affairs reported in April 2016 that 60 million people across Africa, Asia, the Pacific, and Latin America needed food assistance due to weather extremes.
Looking back at 1997–98, the U.N. attributed more than 20,000 deaths and $36 billion in infrastructure damage to that El Niño.
Although El Niño events are complex and evolve differently—as do their impacts and teleconnections—improved predictions would help communities prepare for likely impacts and minimize disruptions. With more advanced warning, resource managers and civic leaders could make adjustments to how they manage fisheries, which crops to plant, what resources to allocate to combat mosquitoes, and when to raise awareness of risks such as fire or mudslides.
By Michael Carlowicz and Stephanie Schollaert Uz.
Design by Joshua Stevens, Lauren Dauphin, Michala Garrison, and Wanmei Liang.
Banner image: Animation of Sea Surface Height Anomaly in the Pacific starting January 1, 2022. Animation by Marit Jentoft-Nilsen, NASA's Goddard Space Flight Center, generated using E.U. Copernicus Marine Service information.
Originally published February 14, 2017; updated October 2, 2024.
Related Reading
- Caviedes, César N. (2001) El Niño in History: Storming Through the Ages. University Press of Florida.
- Grove, R.H. (2007) Global Impact of the 1789-93 El Niño. Nature, 393, 318-19.
- Grove, R.H. (1998) The Great El Niño of 1789-93 and its Global Consequences. The Medieval History Journal, 10, (1-2) 75-98.
- Kessler, William, via NOAA Pacific Marine Environmental Laboratory (2003) Frequently (well, at least once) asked-questions about El Niño. Accessed October 2, 2024.
- NASA’s Jet Propulsion Laboratory (2023) El Niño/La Niña Watch & PDO. Accessed October 2, 2024.
- Nash, J. Madeleine (2002) El Niño: Unlocking the Secrets of the Master Weather-Maker. Warner Books.
- Quinn, W.H. et al. (1987) El Niño occurrences over the past four and a half centuries. Journal of Geophysical Research-Oceans, 92, (C13) 14449–14461.
- Slate (2011, August 24) Weather and War. Accessed October 2, 2024.
- Wikipedia El Niño. Accessed October 2, 2024.