7 min read
Ultrasensitive Far-Infrared Bolometers for Space Astrophysics
The far-IR is a powerful but relatively unexplored spectral band that can enable study of the birth and infancy of galaxies. NASA missions with super-cooled telescopes have the potential for orders of magnitude improvement in sensitivity, but detector improvements are needed to fully capitalize on these new platforms. A JPL team is combining micromachining and superconductivity technologies to make the world’s most sensitive far-IR bolometers.
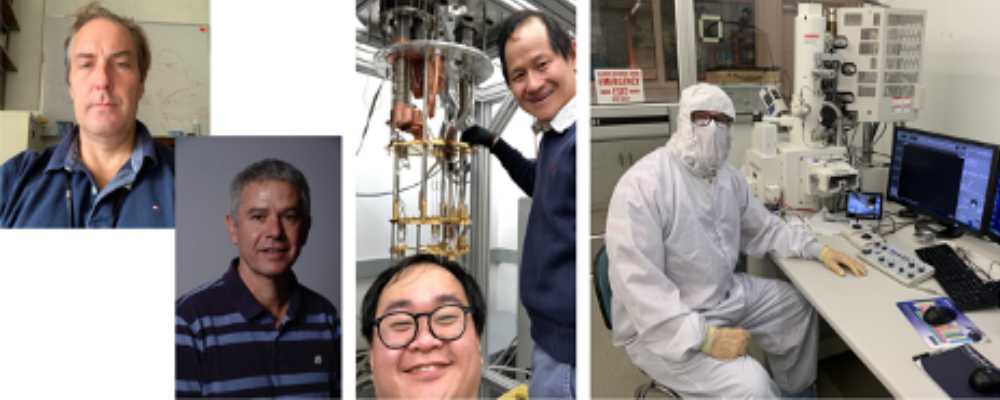
Superconducting detectors are the key to unlocking the secrets of the universe hidden outside the visible band.
The universe is approximately 13.7 billion years old, and astronomers know that galaxies have existed for the vast bulk of this time, at least the last 13.3 billion years. Beginning as small dwarf systems, galaxies have grown, evolved, and merged over time to create the variety we see today. The interior of galaxies is where much complexity in the universe arises: it is where stars form, evolve, and die, forging and distributing the elements necessary for planets, chemistry, and life.
Most of our information about the history of galaxies’ contents is based on measurements in the visible spectral band, for which Earth’s atmosphere is transparent and commercially produced detectors operate with good sensitivity. However, in the last two decades, initial measurements in the far-infrared (or far-IR, defined here as light with wavelengths between 20 to 500 microns) collected by NASA’s Spitzer and the European Space Agency’s (ESA) Herschel space telescopes have shown us that across cosmic time, most of the power from the active interiors of galaxies has emerged in the far-infrared, not the visible waveband. This is because galaxies are inherently dusty; dust absorbs the visible wavelength light and re-radiates the energy in the far-IR. The result is that on average, the visible -wavelength signals from galaxies do not originate in the cores of galaxies’ active regions, but in their exterior skins. A meaningful study of the inner workings of galaxies throughout cosmic history must include a technique which overcomes dust obscuration.
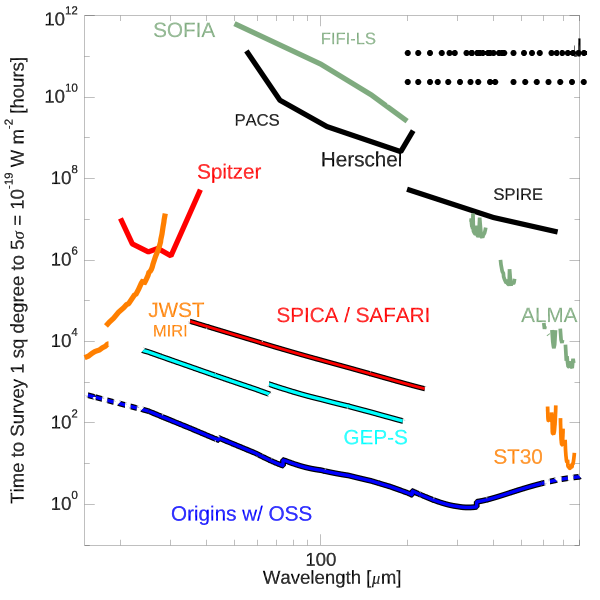
Far-IR spectroscopy may be the best tool nature gives us for this purpose. Far-IR radiation is largely unimpeded by dust, and the spectral features in the far IR both measure the redshifts, thus the look back times (the time it takes light from a distant object to reach Earth), and carry information about galaxies’ heavy-element contents and their stellar and black hole energy sources. While astronomers have demonstrated the use of far-IR spectroscopy for detailed studies of active sites in our own Milky Way galaxy and nearby galaxies, instruments sensitive enough to reach back in cosmic time with spectroscopic measurements were not yet available.
A revolutionary advance is now on the drawing board for NASA’s far-IR astronomers as presented in Figure 1. The key combination is a large cryogenically cooled space telescope and a sensitive far-IR spectrograph. In the far-IR, cooling the telescope to a few degrees above absolute zero is comparable to waiting for the Sun to go down in the visible waveband on a moonless night: the background light which stymies observations goes down by a factor of about a million. NASA’s Spitzer Space Telescope was cooled to below four degrees Kelvin on orbit in its cold phase from 2003 to 2009, and performed flawlessly, demonstrating the basic feasibility of a cold telescope. Spitzer, however, had a modest 85-centimeter diameter mirror, and did not have far-IR spectroscopic capability.
A larger cryogenic telescope presents an engineering challenge, but not a technological one, and a range of mission options are now under study. ESA and the Japan Aerospace Exploration Agency (JAXA) are considering the Space Infrared Telescope for Cosmology and Astrophysics (SPICA) mission featuring a 2-meter telescope and instruments, which could include US participation. In the US, NASA has commissioned studies of both a probe-class mission--the 2-meter Galaxy Evolution Probe—and a 5.8-meter flagship called Origins. All of these concepts are now under consideration by the panels developing the National Academies of Science, Engineering and Medicine’s 2020 Astrophysics Decadal Survey.
The only technological impediment to sensitive far-IR space astrophysics is detector performance. Unlike the visible and even the near-infrared bands, in the far-IR there are few commercial or industrial applications, so detector systems must be developed entirely by the science community. Far-IR detector systems do exist and have been used in a limited fashion by the Herschel telescope. However, achieving the potential capability shown in Figure 1 would require detector arrays with pixels that are 100-1000 times more sensitive than those flown previously, and the most ambitious concepts like Origins would require arrays consisting of 10-100 times more pixels.
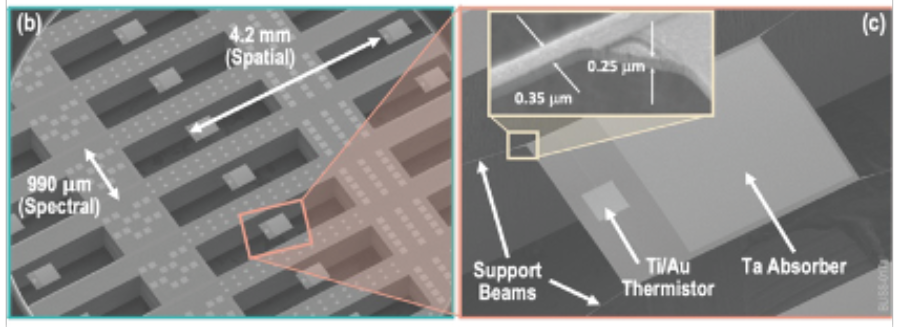
A group at JPL is working to meet these requirements using superconducting detectors. Basic thermodynamic considerations require that any sensitive far-IR detector be cooled to below one degree Kelvin, prohibiting the use of most semiconducting electronics that could be employed for multiplexing and amplification. Superconducting electronics are a natural alternative, given the availability of low-noise superconducting quantum interference device (SQUID) current amplifiers, and the ability to produce high-quality radiofrequency and microwave resonators in thin film superconducting metal—a technology also used in quantum computing experiments. By coupling each detector pixel to a single resonator, and arraying resonators at different frequencies, a single RF or microwave readout circuit can access up to 1000-2000 pixels, each independently biased and read out with a its own Alternating Current (AC) tone. Operating many (50-100) such readout circuits in parallel enables about 100,000 total pixels—the number envisioned by the Origins mission concept.
The JPL team is developing superconducting transition-edge-sensed (TES) bolometers that both meet the Origins sensitivity requirement and use this readout approach. A bolometer consists of an island thermally isolated from a base, which is cooled to a stable low temperature a fraction of a degree above absolute zero. As the schematic in Figure 2 shows, light absorbed on the island elevates the temperature of the island above that of the base, and the temperature is measured with a temperature-sensitive resistor. Bolometers have been used for decades in astrophysics and cosmology, and in the last 15 years, virtually all bolometers use a superconductor that is biased on the edge of its superconducting transition as the temperature sensitive resistor, giving rise to the name TES bolometer. Such devices are the state-of-the-art detectors for ground-based microwave background experiments as well as X-ray spectroscopy.
To improve the sensitivity of the bolometer for far-IR space applications, its electrical response to absorbed power must be increased, which requires a smaller thermal conductance as well as a lower temperature than used in previous experiments. To minimize the thermal conductance, the JPL team uses the approach shown in Figure 2; an island of silicon nitride is suspended with four long and narrow legs from the silicon wafer substrate.
In addition to coupling the bolometers to a resonator-based readout system, the JPL team has made a number of improvements in the devices themselves in the last year, which are now yielding fruit in initial measurements. First, they developed a titanium-gold superconducting bilayer technology to create the temperature-sensitive resistor. By adjusting the ratio of titanium and gold, team members can tune the transition temperature reliably, and have found that the devices have a sharp transition generally (Figure 3, left).
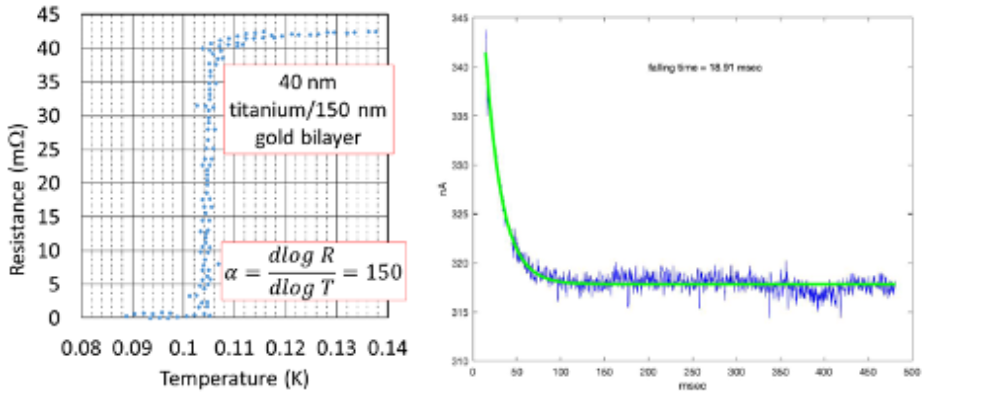
Second, the team developed a wet-release fabrication method to eliminate stray heat capacities and thermal conductances that have plagued similar devices in the past. A wet release is a major challenge for micromachining these delicate structures, but the JPL fabrication process shows high yield (>90%) in subarrays like those shown in Figure 2. Moreover, measurements using the new frequency-based readout indicate these devices are ten times faster than those of the previous generation and meet the requirements for mission concepts under consideration, such as SPICA. The thermal conductance of these new devices also appears to be below the sensitivities required for Origins that are shown in Figure 1. The JPL team plans to confirm these measurements and conduct additional sensitivity measurements as well as a frequency-domain multiplexing demonstration in the coming year.
The research was carried out at the Jet Propulsion Laboratory, California Institute of Technology, under a contract with the National Aeronautics and Space Administration (80NM0018D0004).
Dr. C. Matt Bradford, NASA Jet Propulsion Laboratory, California Institute of Technology
Astrophysics Division’s Cosmic Origins and Strategic Astrophysics Technology Programs
Read more Technology Highlights