PROJECT
Microwave superconducting quantum interference device (SQUID) multiplexing technology development for future x-ray observatories
SNAPSHOT
A new effort that combines two multiplexing techniques will help enable the large-format spectroscopic detector arrays needed for future x-ray astrophysics missions.
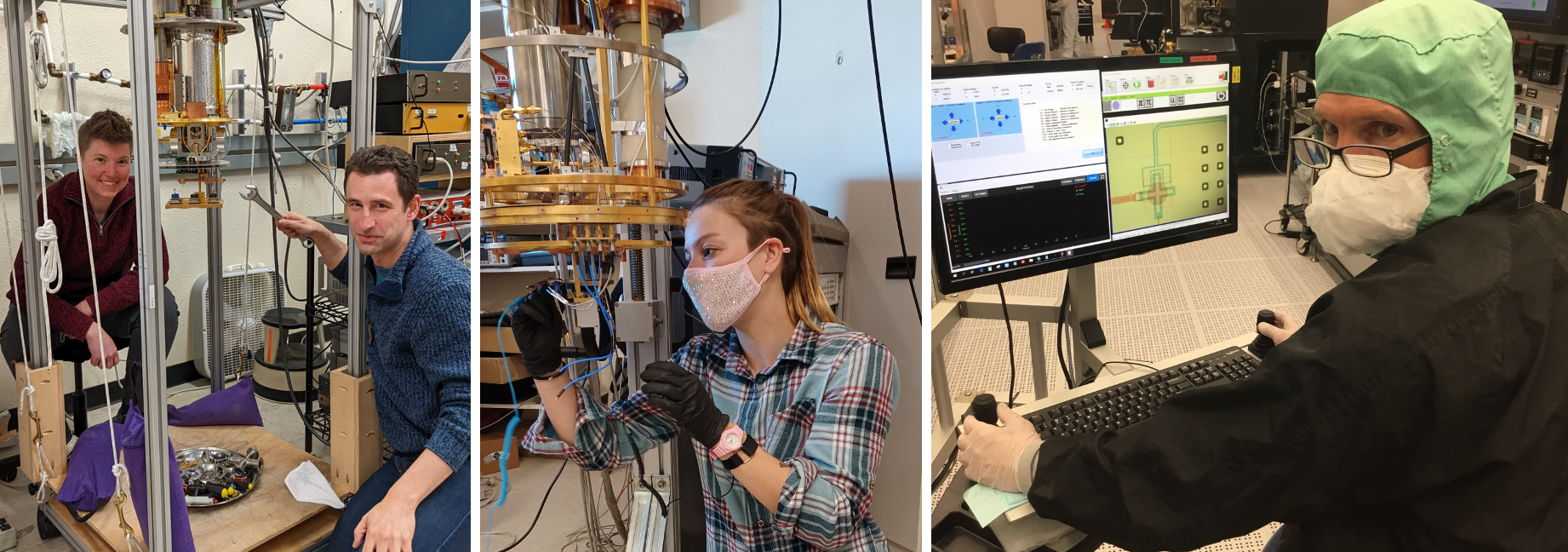
By combining recent technological advances in both x-ray mirrors and spectroscopic x-ray detectors, future x-ray observatories will have the power to transform our understanding of the cosmos. X-ray observations are important because the regions of the Universe with the most active energy release are best observed in the x-ray regime. Spectroscopic x-ray observations are critically important to understanding the drivers of galaxy formation and evolution and comprehending the energy processes of stellar formation. Microcalorimeters are an ideal detector to collect these observations due to their high efficiency and good spectral resolution. Microcalorimeters detect photons by converting the energy of the photon into heat and carefully measuring the temperature increase. When cooled to temperatures below 100 millikelvin (-273.05 degrees Celsius), microcalorimeters can measure the energy of a single x-ray photon with an error of less than one part in a thousand.
To achieve these extremely cold temperatures on a fixed power budget, all aspects of the instrument need to be carefully designed to limit the heat flow from warmer components to the sensors. For example, heat could potentially flow from the warm readout electronics to the sensors via wires connecting these two components. To reduce the heat flow, the number of connecting wires must be limited by combining the signals from many sensors into fewer wires. This process of combining signals into a shared communication channel is known as multiplexing. Multiplexing is fairly common in everyday life: e.g., many different cable TV channels enter your home via a single cable.
The National Institute of Standards and Technology (NIST) Quantum Sensors Group (QSG) has pioneered the use of superconducting quantum interference devices (SQUIDs) to multiplex signals from high signal-to-noise cryogenic detectors before they are sent to warm readout electronics—thereby reducing the number of connecting wires required and limiting potential heat transfer. The QSG is currently collaborating with the x-ray microcalorimeter group at NASA GSFC to integrate one type of multiplexing (time-division multiplexing) into the 3,000 spectroscopic sensor array for the X-ray Integral Field Unit of the Athena Satellite Mission.
Even more sensors are required to meet science goals of future x-ray satellite missions. For example, the science goals of the Lynx X-ray Observatory – a large mission concept selected for study for the 2020 Decadal Survey on Astronomy and Astrophysics, along with the HabEx, LUVOIR, and Origins mission concepts – require the microcalorimeter instrument’s focal plane to have over 100,000 pixels. Multiplexing the readout of such a large array is a significant technological challenge. The most promising strategy to achieve the required multiplexing factor is to use a combination of thermal and electrical multiplexing.
The thermal multiplexing of microcalorimeters was pioneered by the x-ray microcalorimeter group at NASA Goddard Spaceflight Center and is achieved by attaching multiple x-ray absorbers to each microcalorimeter sensor with different lengths of wire to create different levels of thermal conductance. The pulse shape for an absorbed photon changes according to the different thermal conductance levels and thus the pulse shape can be used to identify which pixel absorbed the photon. A microcalorimeter with this type thermal multiplexing is referred to as a hydra, after the mythical many-headed serpent.
Electrical multiplexing is achieved via microwave SQUID multiplexers (mMUXs) that transform the baseband signals from the microcalorimeter sensors into frequency shifts at gigahertz frequencies. This multiplexing architecture takes advantage of the large bandwidth microwave components can use to combine signals from thousands of sensors on a single coaxial cable.
The NIST QSG team, along with researchers from the University of Colorado Boulder, has designed, fabricated, and tested microwave SQUID multiplexers that are optimized to operate on the readout of thermally multiplexed microcalorimeters. These microwave SQUID multiplexers were targeted to meet the requirements proposed for the Lynx X-ray Microcalorimeter (LXM) instrument for the Lynx x-ray observatory but are also appropriate for other potential x-ray satellite missions that require large arrays of microcalorimeters.
The team carefully studied the Lynx LXM requirements and produced different microwave SQUID multiplexer chip designs with optimal size, weight, and power for the various types of microcalorimeters in the LXM. A photograph of a prototype microwave SQUID multiplexer chip optimized for LXM is shown above. These prototype chips were fabricated in the NIST Boulder Microfabrication Facility (BMF). The BMF is a 1700 m2 (18,000 ft2), International Standards Organization (ISO) class 5 cleanroom for micro- and nanofabrication with dedicated facilities for the fabrication of superconducting detectors and electronics.
The new microwave SQUID multiplexer protype chips were tested in cryogenic testbeds that are capable of cooling microcalorimeters to 20 mK and have x-ray access to allow confirmation of detector performance using known x-rays lines. A custom sample box, shown in the photograph above, was designed to test microwave SQUID multiplexers employing various designs in conjunction with hydra transition-edge sensor (TES) arrays. Using this box, the team successfully demonstrated microwave SQUID multiplexer operations on the readout from the hydra microcalorimeter. Based on these protype multiplexer test results, the team is currently modifying the SQUID multiplexer design to better match the pulses from the hydra TESs.
NASA SMD funded the NIST QSG to explore these multiplexing technologies through the Astrophysics Division’s Strategic Astrophysics Technology (SAT) Program. The microwave SQUID multiplexing technology developed using this funding was baselined for both the Lynx X-ray Observatory and the Origins Space Telescope mission concepts and is also being considered for other potential missions.
PROJECT LEADS
Dr. Douglas Bennett and Dr. John A.B. Mates, National Institute of Standards and Technology (NIST) and Dr. Kelsey Morgan, University of Colorado, Boulder.
SPONSORING ORGANIZATIONS
APD Strategic Astrophysics Technology Program
Read more Technology Highlights