PROJECT
Extremely Low-noise, High Frame-rate X-ray Image Sensors for Strategic Astrophysics Missions
SNAPSHOT
New imaging technology will help enable future large X-ray telescopes to trace the origin and growth of black holes and the ways they’ve shaped the cosmos.
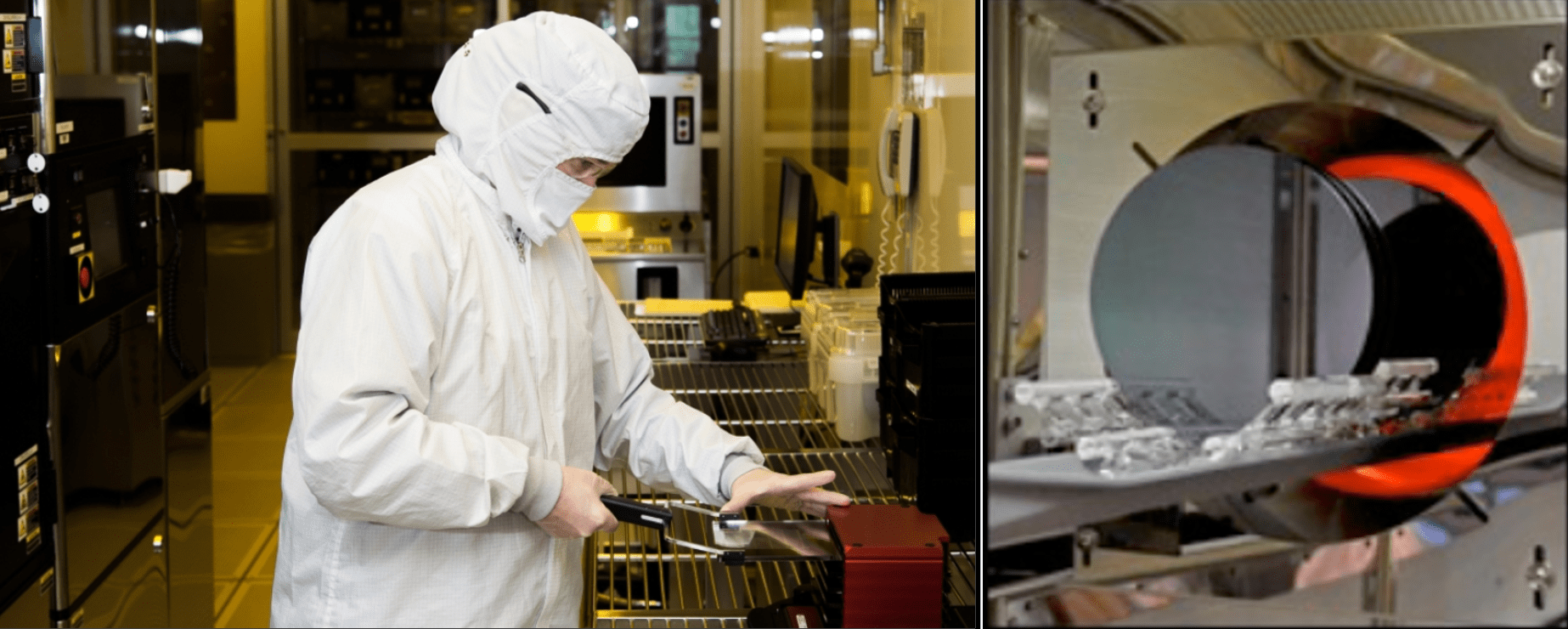
How did our universe of galaxies and stars come to look as it does? NASA’s Great Observatories, including the Chandra X-ray Observatory and the Hubble Space Telescope, have revealed that the interplay between galaxies and the enormous black holes they host is key to answering this question, but precisely how this interaction works remains a mystery. For example, how did black holes a billion times the mass of the Sun grow so soon after the big bang? How do black holes grow in apparent lockstep with their host galaxies? How do black holes control star formation over distances many billion times their own size?
Just as the current Great Observatories were instrumental in raising these questions, a new generation of facilities—including X-ray telescopes with much larger collecting areas and much faster and more sensitive imaging sensors—is needed to answer them. NASA has been developing technologies to enable these advances for the past decade.

X-rays are emitted by almost all objects in the cosmos—from planets and stars to galaxies and the giant black holes they host. X-ray astronomy is especially important for understanding the latter since the matter closest to a black hole is a powerful source of X-rays. X-ray photons carry a thousand times as much energy as photons of ordinary light we can see, so specially developed X-ray sensors are needed to record them. These sensors can not only sense X-ray photons but also measure their energies (analogous to the colors of visible light).
Next-generation X-ray telescopes will collect photons 10-30 times faster than those operating in space now. To take full advantage of this capability, the sensors on these observatories must at a minimum be able to record and process individual photons at correspondingly faster rates. Even faster sensors are desirable since they would yield a clearer picture of X-ray sources changing over time. Moreover, the new observatories need to characterize fainter and more distant cosmic X-ray sources than any we have yet observed. Photons from these sources will appear to have correspondingly lower energies (analogous to redder colors), owing to the expansion of the universe, so detecting and measuring the energies of these photons requires sensors with exquisite sensitivity and very low internal noise. Finally, these advanced capabilities must meet the stringent constraints on size, mass, and power consumption imposed on spaceflight instrumentation.
To meet these challenges, a team of researchers at the Massachusetts Institute of Technology (MIT) Kavli Institute (MKI) for Astrophysics and Space Research, MIT Lincoln Laboratory, and the Stanford University Kavli Institute of Particle Astrophysics and Cosmology is leveraging almost forty years of NASA investment in development and operation of X-ray sensitive charge-coupled devices, and applying the latest developments in micro-fabrication and microelectronics technologies to obtain the required performance gains. Their goals are to increase sensor speed by a factor of 100 and reduce noise by a factor of three while maintaining power consumption at roughly the same level required by current technology.
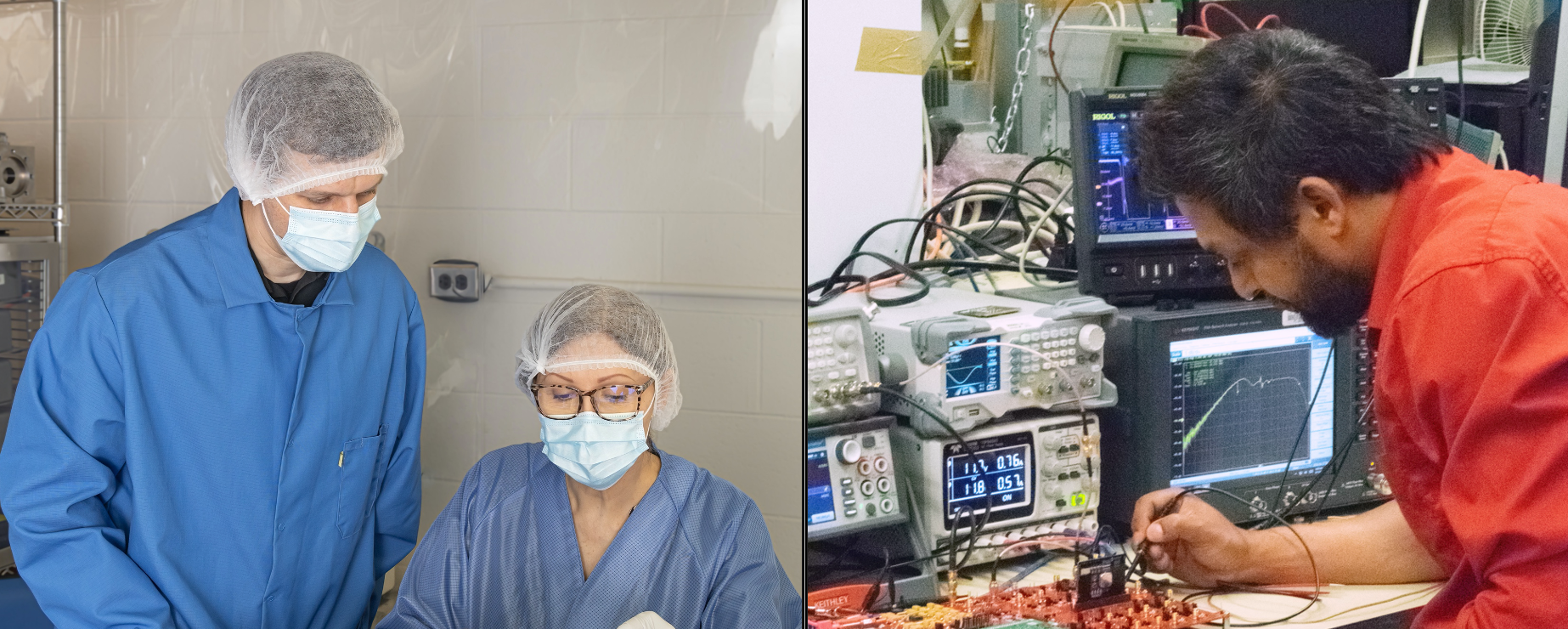
Three technical innovations put these goals within reach. First, new sensor amplifier structures that operate with low noise at much higher speed than those on Chandra have been demonstrated in the laboratory, and architectures with potential for even lower noise are being tested. Second, advances in the processes used to build X-ray sensors permit lower operating voltages and thus enable lower-power operation. Finally, the team is developing application-specific integrated circuitry (ASIC) to provide fast, low-noise, analog signal processing tailored to the sensor amplifier characteristics using a fraction of the power required by discrete processing electronics.
Researchers at MKI have tested prototype sensors designed and fabricated at MIT Lincoln Laboratory that demonstrate noise levels better than the X-ray sensors on Chandra (less than 3 electrons in each image pixel)—all while operating 20 times faster and with no increase in power per unit detector area. The team’s Stanford members have designed an 8-channel ASIC with a projected noise contribution of just over 1 electron per pixel and per-channel data rates up to 5 million pixels per second. By combining these developments, it becomes feasible to design and build X-ray sensors capable of high-speed, low-noise imaging at rates 100 times faster than Chandra’s.
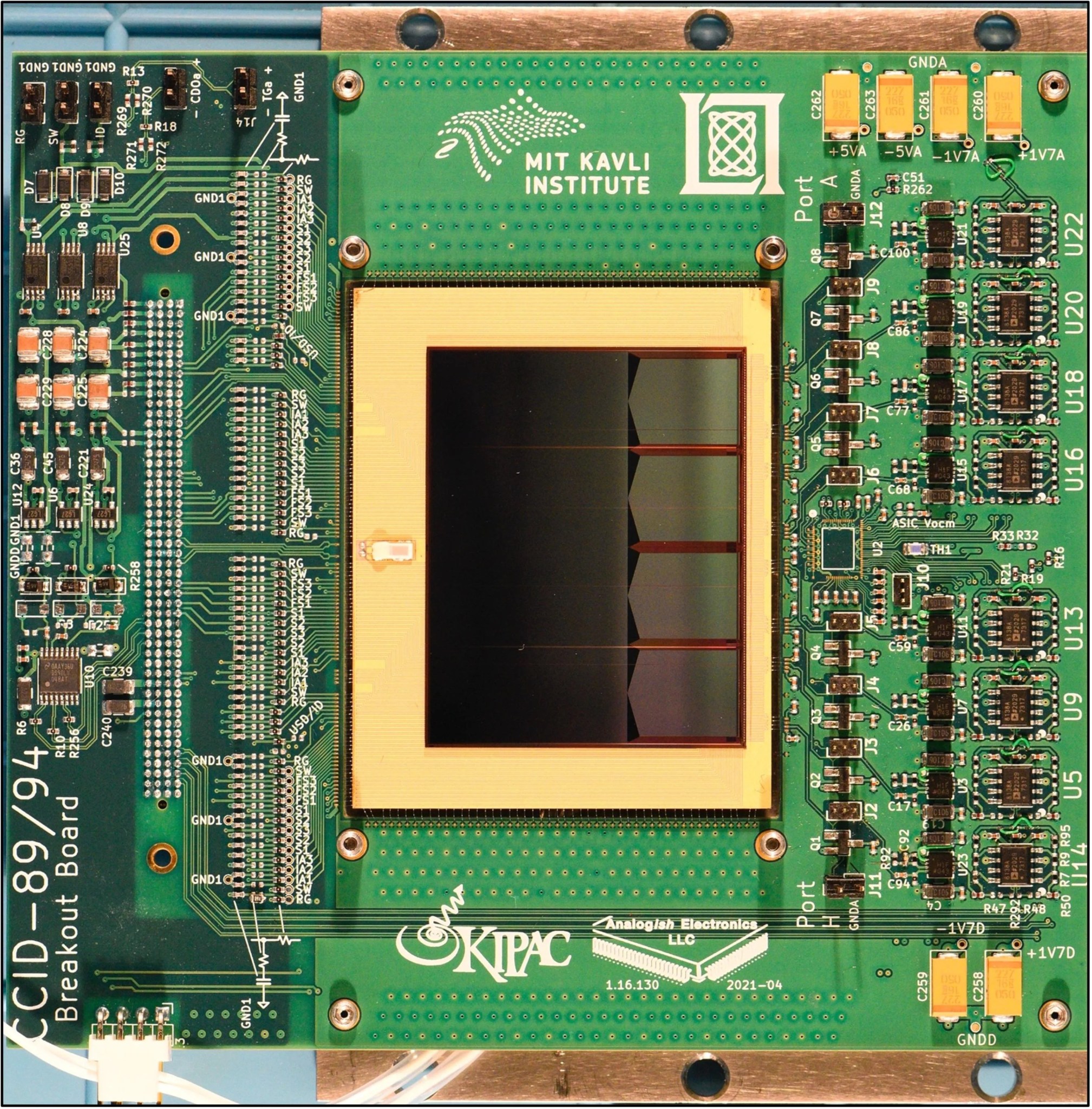
According to Dr. Bautz, “We have been privileged to work with the NASA SMD Astrophysics Division and MIT Lincoln Laboratory to develop and deploy imaging technology for a number of successful astrophysics missions, and to have participated in scientific research those missions have enabled. This project may be our most ambitious sensor development yet, and we’re excited to help realize even more ambitious X-ray missions.”
NASA’s Astrophysics Division has been investing in fast X-ray imaging sensor development since the mid-2000s. The technologies described here are under consideration for a future X-ray probe and a subsequent flagship X-ray Observatory, and potentially other future missions.
PROJECT LEADS
Dr. Marshall Bautz, MIT Kavli Institute (Principal Investigator); Dr. Christopher Leitz, MIT Lincoln Laboratory (lead Co-Investigator); Prof. Steven Allen, Stanford University (lead Co-Investigator).
SPONSORING ORGANIZATIONS
NASA Astrophysics Division Strategic Astrophysics Technology (SAT) Program, with additional support from the MIT Kavli Institute for Astrophysics and Space Research, the Stanford Kavli Institute for Particle Astrophysics and Cosmology, and The Under Secretary of Defense for Research and Engineering.