9 min read
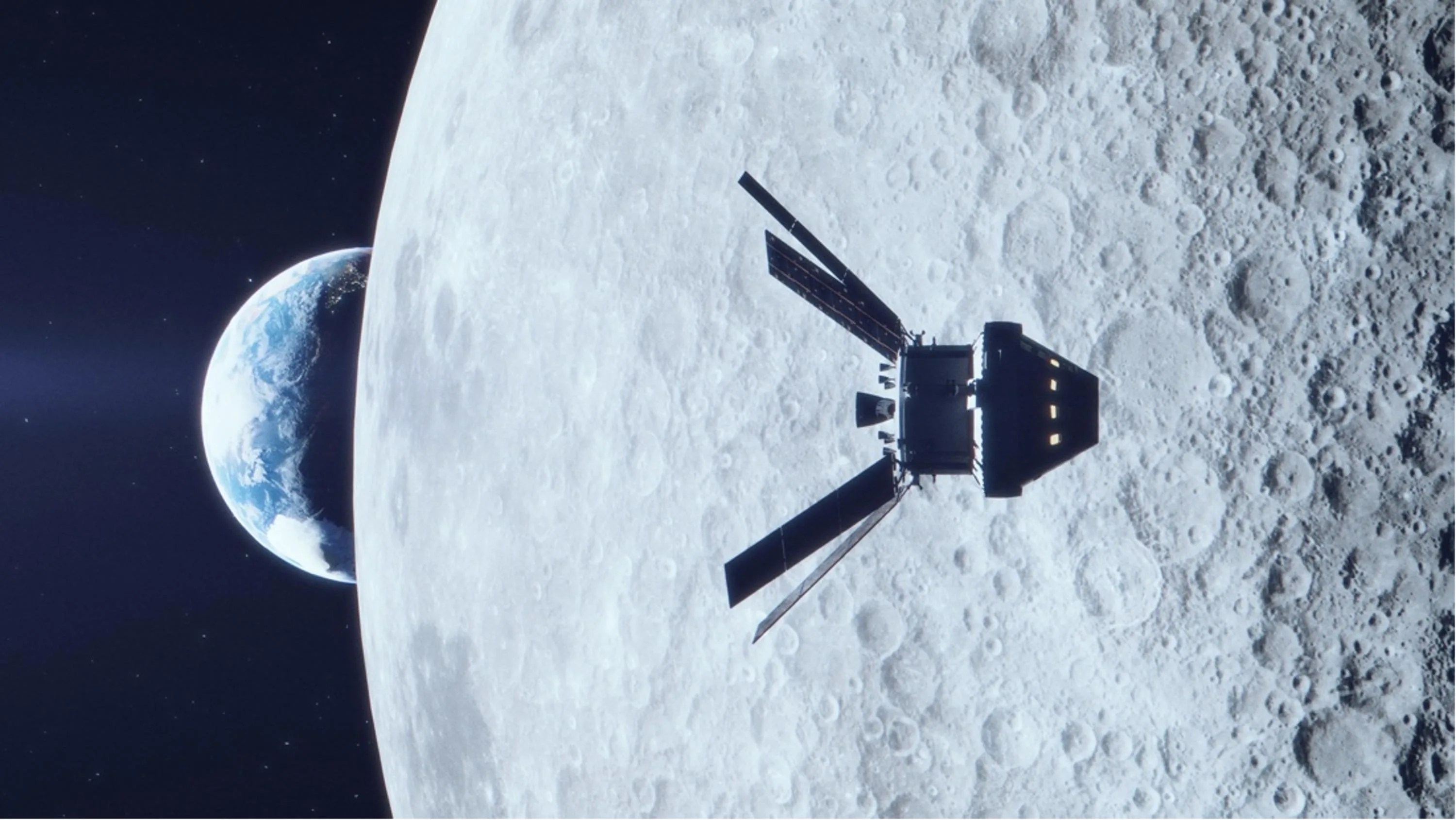
When gazing up at the Moon in the night sky, envision a future where humans can live and work on the lunar surface. Part of that work includes valuable research, as the Moon offers two environments important for science – and to which we do not currently have access down on the ground: The Moon’s gravity is only about one-sixth of Earth’s, and it has a very thin atmosphere which is exposed to more intense radiation than exists on Earth. Additionally, establishing a sustainable presence on the Lunar surface is an essential step towards preparing for missions to Mars.
However, before that, to go farther and stay longer in space, including to Mars, we must investigate how biological and physical phenomena behave in the unique environment of the Moon.
To pave the way for human exploration of the Moon, Mars, and beyond, NASA’s Artemis missions are set up as a series of increasingly complex missions. The first, Artemis I will be an uncrewed test flight of the Space Launch System (SLS) and the Orion spacecraft around the Moon. The primary goals for this test flight are to demonstrate Orion’s systems and thus ensure a safe journey to the Moon and back for the first crewed flight on Artemis II.
Though uncrewed, the crew compartment of Artemis I will have minuscule biological life forms aboard, along with other scientific payloads. Some of these tiny travelers aboard Orion make up NASA’s Biological and Physical Sciences’ Biological Experiment 01 (BioExpt-01) mission which will serve as a pathfinder for biological research beyond low-Earth orbit.
BioExpt-01 includes four investigations that will evaluate effects of the deep space environment on the nutritional value of plant seeds, DNA repair within fungi, adaptation of yeast, and algal gene expression. By sending these investigations through two giant donuts of radiation around Earth called the Van Allen Belts — to areas beyond low-Earth orbit — researchers will gain understanding of the elevated levels and impacts of ionizing radiation on biological life forms like never before. This knowledge will help us to understand how biological life forms can better thrive in deep space and support future manned missions to the Moon and Mars.
Sending humans back to the Moon and eventually to Mars requires seeking answers to fundamental questions. Since humanity last stepped on the Lunar surface on December 19, 1972, scientists have been wondering how deep space impacts the human body and other life forms, like plants, which will sustain humans for long duration space missions.
Four studies within BioExpt-01 using model organisms and plant seeds aim to help answer critical questions essential to ensuring crew health on future long-duration missions:
The Deep Space Radiation Genomics investigation will pioneer scientific discovery by correlating which genes provide cells with a higher probability of survival as well as understanding the types and doses of radiation they experience beyond Earth’s protective magnetosphere.
“We’re using yeast cells in this investigation because they share a lot in common with our own human cells,” explains Luis Zea, principal investigator at the University of Colorado in Boulder, Colorado. “Rather than sending limited numbers of humans in a spacecraft, we can send millions of yeast cells in a tiny bag and study deep-space effects on those cells, which would be very similar to what would occur within our own cells under the same conditions.”
To prepare for this flight, Zea and his team had to develop a new type of hardware that allows scientists to do their research in space without the presence of human travelers within the spacecraft.
“With Artemis I, we need to have the capability to start the experiment at a specific time during the flight,” said Zea. “So we developed new hardware called PLASM – that’s short for Peristaltic Laboratory for Automated Science with Multigenerations – at BioServe which will be able to detect where it is in relation to Earth and the Moon. It will autonomously start the experiment once it passes through the last Van Allen Belt into a high -radiation environment in order to study how deep space radiation affects the DNA and how the DNA is repaired by these cells.”
It’s essential to understand the damage deep-space radiation does to DNA and which DNA repair mechanisms are more efficient under these conditions. This knowledge will serve as the baseline for future studies on how researchers can support reliable DNA repair mechanisms for astronauts traveling to deep space.
Traveling to deep space and back requires an ample amount of fuel, oxygen, and nutrition. As it is, available room on board a rocket is hard to come by, so it raises the question: What is portable and can accomplish all three needs?
The Fuel to Mars investigation will aim to identify genes and metabolic pathways in the green unicellular alga Chlamydomonas reinhardtii that appear to have enhanced survival advantages during exposure to the combined effects of space radiation and altered gravity. These could be incorporated into parent strains in future studies to optimize generation of hydrogen and other fuels in space.
“As it turns out, algae is a wonderful source of hydrogen, which is a gas, but can be used as a fuel,” said Holly Birdsall, Fuel to Mars co-principal investigator at Baylor College of Medicine in Houston, Texas. “Algae is also edible and can be used to make vitamins or oils that humans need for nutrition. It also couldn’t be simpler to grow — algae requires water and light and that’s just about it. They are a great tiny traveling companion that can answer a lot of our needs.”
This investigation is led by Birdsall and Timothy Hammond, principal investigator at Duke University School of Medicine in Durham, North Carolina. Together, they hope to gain a deeper understanding of how cells interact with radiation and how to best optimize plants in space.
"Individual genes in Chlamydomonas have been knocked out helping elucidate the role of important plant genes in the unique environment of deep space,” says Hammond. “Because of this, we can determine which genes determine survival, making green algae the key to studying cells and their reactions in the entire plant world.”
Investigating Roles of Melanin and DNA Repair on Adaptation and Survivability of Fungi in Deep Space will help researchers understand how cells adapt to high levels of cosmic radiation during space travel by focusing on fungi’s natural protective qualities such as DNA repair and melanin synthesis.
Zheng Wang, principal investigator at the Naval Research Laboratory in Washington, DC, hopes this investigation will help researchers identify new ways to prevent radiation damage to humans and equipment in space.
“Fungi are one of the first organisms to thrive in environments on Earth that experience nuclear activity, so there’s a lot of interest in how fungi are able to make life more habitable not only terrestrially but also in deep space,” according to Wang. “Astronauts will have to overcome higher radiation levels during space travel, and one possible solution is to use fungal products to protect them.”
The different types of fungi that Wang and his team are studying represent different characteristics that help fungi adapt to similar conditions. The first type is a healthy wild-type – which means it’s normally occurring -- strain. The second and third strains are mutants unable to perform DNA repair, which is important to investigate since DNA carries the instructions for how a body should function. The fourth mutant strain cannot produce melanin, which is a pigment occurring in the hair, skin, and iris of the eye in humans and animals which helps block out damaging UV rays.
Wang and his team will compare the survival of these strains to understand how important DNA repair and melanin are to thriving in deep space. Their results will help engineer treatments to protect astronauts from high levels of radiation during deep space exploration, and help establish a sustainable presence in space, on the Moon, or on Mars.
Life Beyond Earth: Effect of Spaceflight on Seeds with Improved Nutritional Value investigation will study the impact of space-flight beyond the Van Allen radiation belt on amino acids (the building blocks of proteins) in Arabidopsis thaliana seeds.
As the main component of skin, muscles, organs, bones, and nails, protein makes up a significant part of the human body and is present in every cell. These proteins are made up of amino acids, which are essential for human diets.
When plants and astronauts travel to space, they are subject to several stressors. Plants have been found to lose nutrients, including amino acids, in microgravity. To travel long distances, such as to Mars, plants need to produce more nutrients in a confined space with limited resources.
“Our technology equips plants with a higher content of amino acids that are essential for the human diet,” said Federica Brandizzi, principal investigator for the investigation at the College of Natural Science at Michigan State University, in East Lansing, Michigan. “We’re attempting to see how we can improve plant productivity using plants that we’ve modified to have more resources to withstand spaceflight.”
Brandizzi and her team will send seeds of a model plant called Arabidopsis thaliana, which have been genetically engineered to produce high levels of specific essential amino acids. They will study how deep space affects the levels of amino acids within these genetically modified seeds and see if they confer a competitive advantage for germination and produce stronger plants.
Related Articles
- Artemis I - https://www.nasa.gov/artemis-1
About NASA’s Biological and Physical Sciences
NASA’s Biological and Physical Sciences Division pioneers scientific discovery and enables exploration by using space environments to conduct investigations both on Earth and in Space. Studying biological and physical phenomenon under extreme conditions allows researchers to advance the fundamental scientific knowledge required to go farther and stay longer in space, while also benefitting life on Earth.