6 min read
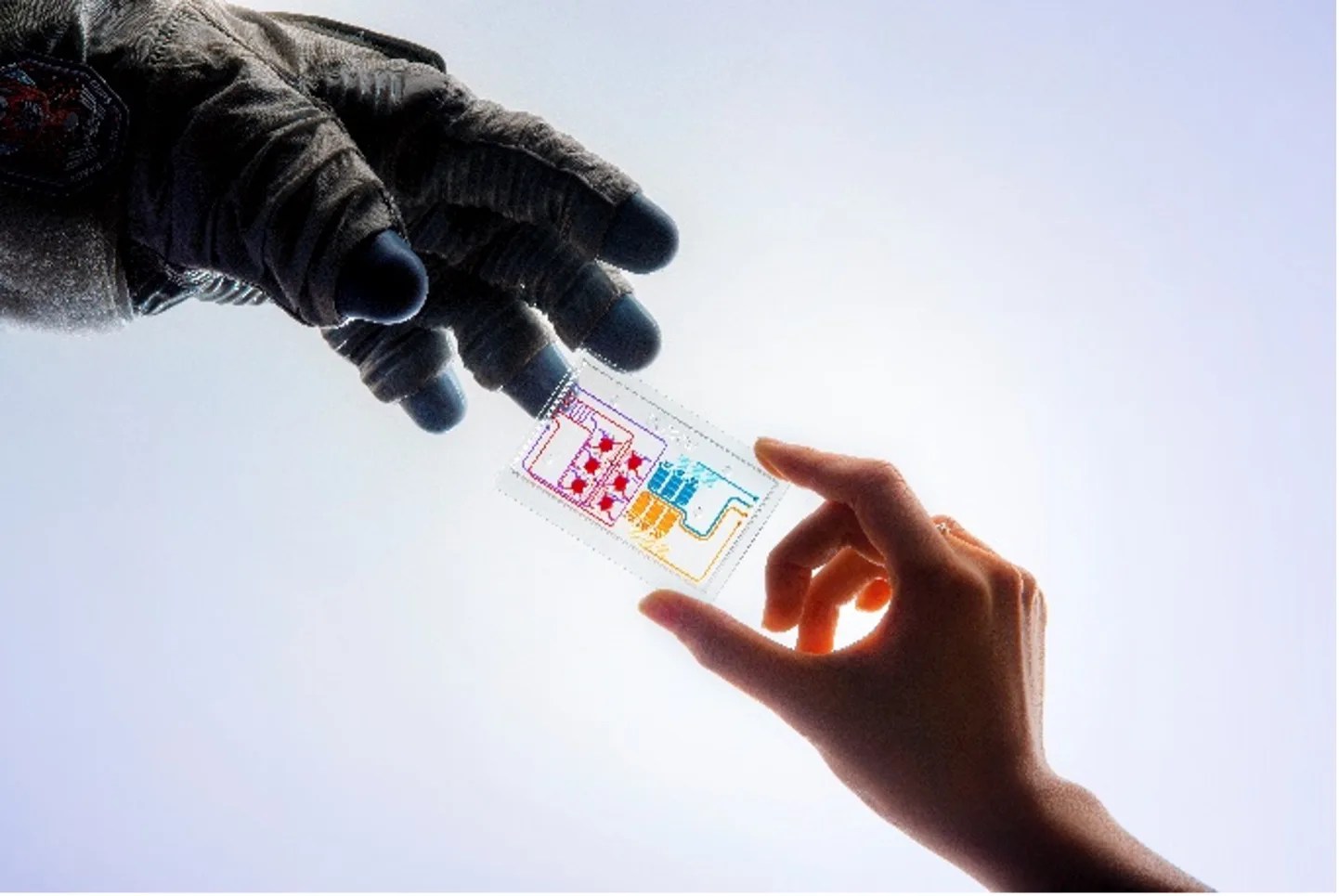
Science fiction or reality? Before traveling to Mars, NASA needs to learn how spaceflight is going to affect the astronauts on this demanding journey – and now they can thanks to miniature avatars.
These avatars will be able to model diseases and help determine medications that are personalized for the individuals who will embark on the uncharted journey. Astonishingly, this remarkable technology exists today and is not a work of science fiction.
Space travel is exceptionally challenging – particularly on the human body. In spaceflight, astronauts experience a number of stressors like bone loss, muscle atrophy, immune system changes, and other disorders that can affect their health.
Collectively, these stressors can contribute to health issues in astronauts, and as humanity continues to explore space further and longer than before, these astronauts will not have access to a fully equipped hospital or lab to treat their ailments. Enter the need for disease models, advanced drug development and methods to understand biological changes in deep space prior to the voyage.
The first 3D tissue chip was introduced in 2010 and, after extensive research over the past 12 years, has shown promising results. These chips are engineered to be roughly the size of a credit card and can be used to represent various human organs by modeling both structure and function of different parts of the body – such as the lungs, liver, kidneys, intestines, bone, muscle and heart. Combined, they can represent multi-organ human systems.
These miniature avatars of living organ tissues are engineered using a person’s blood or skin cells converted through induced pluripotent stem cell (iPSC) science to grow the tissue of interest on microfluidic chips. Researchers use these tissue chips within labs, both on Earth and in space aboard the International Space Station, to gain deeper insights into biological effects from spaceflight exposure, understand disease modeling and to predict the efficacy of potential pharmaceuticals.
“3D tissue chips mimic biological organs by providing multi-layer structures and environments that are representative of what you would find inside of the human body,” says Dr. Lisa Carnell, Program Scientist for Translational Research in NASA’s Biological and Physical Sciences division.
3D tissue chips have already proven their value in current research, so scientists have high hopes for this new technology in the future. Recently, tissue chips were used to study COVID-19 helping to understand the disease pathology and to test potential therapeutics.
NASA’s Biological and Physical Sciences division, the Department of Health and Human Services National Institutes of Health (NIH), Biomedical Advanced Research and Development Authority (BARDA), and FDA are collaborating on the next steps in advancing 3D tissue chips even further.
Currently, 3D tissue chips are only viable for about a month limiting their use. This multi-agency collaboration aims to change that by funding nine contracts that will extend tissue viability and physiological function to a minimum of six months. By extending tissue viability, researchers will be able to test acute and chronic exposures to drugs, radiation, environmental hazards, infection, and microgravity, to name a few.
“NASA wants to send these avatars to deep space, the Moon, and Mars, so that we can understand what is happening to human biology in space and come up with ways to protect astronauts,” explains Carnell. “These are going to be really valuable for NASA, to ensure that humans can thrive in deep space on future Artemis missions.”
Here are the teams of researchers working to extend the tissue chip longevity to study a wide variety of biological changes including neurotoxic stressors, radiation exposure, and acute and chronic exposures to drugs to name a few.
Elizabeth Blaber, Rensselaer Polytechnic Institute, Understanding the Brain-Liver-Gut Axis during Spaceflight and Aging investigation will provide long-term, multicellular complex human tissue models for studying responses to acute and chronic spaceflight stressors that mimic deep space exploration.
Joel Blanchard, ICAHN School of Medicine at Mount Sinai, Identification of Biomarkers and Pathological Mechanisms via Longitudinal Analysis of Neurological and Cerebrovascular Responses to Neurotoxic Stress Using a Multi-cellular Integrated Model of the Human Brain investigation will determine how genetically diverse human brain tissue responds to neurotoxic stresses that may be encountered in space.
Guohao Dai, Northeastern University, Bioengineer Long-lasting 3D Neurovascular Microphysiological System to Model Chronic Inflammation-mediated Neurodegeneration investigation will develop a long-lasting neurovascular model that can be used to evaluate the impact of chronic stressors to the brain.
Dr. Guillermo García-Cardeña, Brigham and Women’s Hospital, Inc. Modeling Anthracycline-triggered Vascular Dysfunction investigation will use an organ-on-chip platform to investigate how a chemotherapy drug, doxorubicin, triggers vascular cell dysfunction which will support development of new therapeutic interventions to curtail off-target effects.
Abhishek Jain, Texas A&M, Long-term Patient iPSC Vessel Chip Model to Assess Stressors of Atherosclerosis and mRNA Therapeutics investigation will study vascular signaling mechanisms to identify therapeutics for aging and radiation-related vascular diseases.
Christopher Porada, Wake Forest University, Long-lived Single- and Multi-organ Tissue Equivalent (OTE) Platforms to Model the Response of Human Tissues to Various Stressors investigation will use human organ tissue equivalents (OTEs) to gain insight into how different human tissues respond to spaceflight.
Gordana Vunjak-Novakovic, Columbia University, MORPH: Multi-Organ Repair Post Hypoxia investigation will study the effects of acute hypoxia on organ systems that are most critically affected: brain, heart, bone marrow and vasculature.
Joseph Wu, Stanford University, Assessing Long-Term Effects of Radiation Exposure in Engineered Heart & Vascular Tissues investigation will gain a better understanding of how and why radiation exposure causes disease by using heart and blood vessel-like tissues created with stem cell biology and tissue engineering technology.
Catherine Yeung, University of Washington, Extended Culture of Kidney MPS and Organoids to Model Acute and Chronic Exposure to Drugs and Environmental Toxins investigation will use kidney chips and organoids to better understand injury and recovery from chronic exposure to drugs, toxins and pathogens.
NASA’s Biological and Physical Sciences Division pioneers scientific discovery and enables exploration by using space environments to conduct investigations not possible on Earth. Studying biological and physical phenomenon under extreme conditions allows researchers to advance the fundamental scientific knowledge required to go farther and stay longer in space, while also benefitting life on Earth.