PROJECT
The Microwave Electrojet Magnetogram (MEM)
KEY POINTS
The low SWaP MEM sensor enables cost-effective implementation of future high-impact ionospheric current investigations on resource-limited missions, including CubeSat constellations.
Understanding the interaction and coupling processes within Earth’s atmosphere, ionosphere, and magnetosphere system is one of the key scientific challenges identified in the Decadal Strategy for Solar and Space Physics (Heliophysics) in 2012. Observations of ionospheric electrojet currents flowing around 105-km altitude are crucial to this endeavor but have been thus far unobtainable. These currents are part of a vast current system flowing between the magnetosphere and the ionosphere and the lack of measurements have left many fundamental questions unanswered despite several decades of research. NASA is currently developing a new remote sensing instrument to cost-effectively provide these critically needed measurements.
Over the past year, NASA’s Microwave Electrojet Magnetogram (MEM) project has been maturing a novel low size, weight, and power (SWaP) array receiver capable of remotely imaging the ionospheric current structure from an orbital platform. Each element of this MEM sensor spectrally resolves the three Zeeman-split O2 thermal lines at 118 GHz and provides measurements of the current-induced magnetic field globally at multiple locations simultaneously under all solar and atmospheric illumination conditions. Traditionally, these ionospheric current strengths have been inferred from magnetic field variations detected by ground-based magnetometers below the currents and spaceborne magnetometers high above the currents. The measurements from these methods, however, are either spatially sparse (from the ground) or smeared with degraded spatial resolution (from space). The groundbreaking MEM measurements will overcome these limitations and provide the first-ever, simultaneous magnetic field measurements at multiple locations and times near altitudes of ~105 km from a single spacecraft.
MEM is a modern microwave receiver system that consists of multiple heterodyne full-Stokes spectro-polarimeters operating at ambient temperature. The front-end of each polarimeter collects the incoming 118 GHz signal and non-linearly mixes it with the signal generated by a reference local oscillator (LO) that is set at a nearby frequency. The mixed output at the different frequencies conveys the amplitude and phase of the original higher frequency 118 GHz signal, but at a lower, more easily processed, carrier frequency. The receiver front-end is technologically mature and can be built compactly—the size of a human thumb—and is thus easily accommodable on CubeSat or SmallSat platforms. A critical challenge of the multi-element MEM concept is the power consumption and the size of multiple spectrometers in the receiver back-end.
The MEM project team, led by Dr. Jeng-Hwa Yee at the Johns Hopkins University, Applied Physics Laboratory (APL), is currently developing a multi-mission compact and low-power Baseband Spectrometer Module (BSM). The architecture of this BSM is scalable to support a varying number and type of sensing elements and thus a variety of mission concepts. The hardware realization is small, low-power and radiation tolerant. The BSM design is based on a flight technology development program at APL, the CoreSat Single Board Computer (SBC). The CoreSat SBC is a 4” by 6” card that can support both general command and data handling, as well as specialized digital signal processing applications.
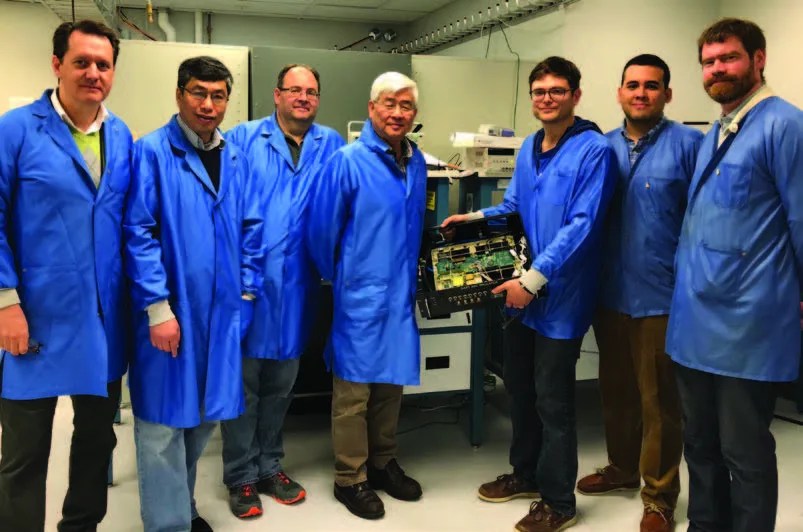
“A single spacecraft ionospheric current mission with a four-element MEM sensor onboard would be equivalent to flying four spacecraft equipped with low-noise magnetometers...” - Dr. Jeng-Hwa Yee
The MEM BSM utilizes a modular and scalable architecture to simplify the CoreSat SBC. Unnecessary components are either removed or reduced in number, yielding a smaller and more power-efficient BSM card that can be mated to a carrier card designed for any specific mission.
MEM has a flexible design so that the number of elements, exact look angles, and combination of polarizations can be tailored to meet the requirements of the desired missions. According to Dr. Yee, “A single spacecraft ionospheric current mission carrying a four-element MEM sensor would be equivalent to flying four spacecraft equipped with low-noise magnetometers and its measurements would not be affected by the distance to the currents.” While the MEM receiver front-end is technologically mature, the ongoing receiver back-end—the BSM development effort—will result in a TRL 6 technology for deployment in NASA’s future heliophysics missions. To further advance the MEM’s TRL, the project is building a BSM prototype and verifying its performance and power consumption.
SPONSORING ORGANIZATION
Heliophysics Division’s H-TIDeS Program
PROJECT LEAD
Dr. Jeng-Hwa Yee, Johns Hopkins Applied Physics Laboratory