Telescopes
Astronomers observe distant cosmic objects using telescopes that employ mirrors and lenses to gather and focus light.
The size of the main mirror or lens determines how well a telescope can collect light. The larger a mirror or lens, the more light it collects, and the better its ability to detect fainter objects.
Refracting telescopes
A telescope using a lens for its main optical element is called a refracting telescope. Like eyeglasses, the lenses bend, or refract, light passing through them. The first telescopes, developed in the 1600s, were refractors, as are many backyard telescopes today.
But very large lenses make refracting telescopes large and heavy, which makes them difficult to use in space.
Reflecting telescopes
A telescope that uses a mirror as its primary optical element is called a reflecting telescope. Large mirrors can be made thinner and lighter than lenses of the same size, which makes reflecting scopes ideal for sending to space. (Backyard telescopes can also have mirrors, too.)
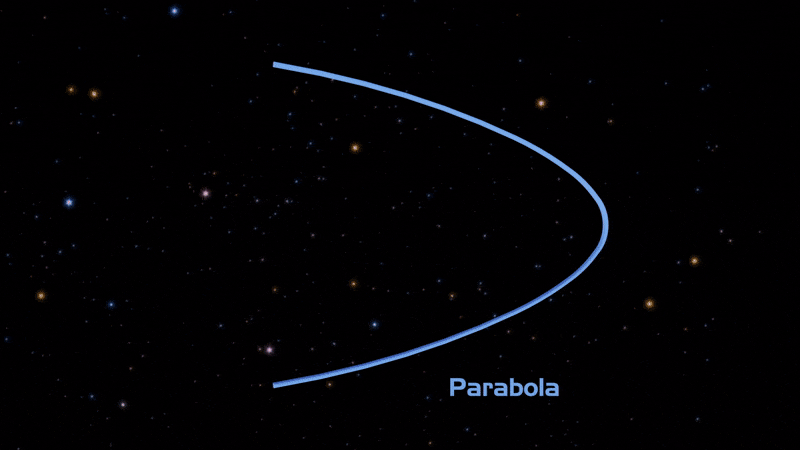
Mirrors for most telescopes are based on the same shape – a paraboloid, a 3D version of a parabola that has been spun around its center and resembles the nose cone of a rocket. Engineers design and craft a telescope’s mirrors based on what it will study using different parts of the paraboloid.
Mirrors for visible and infrared light and dishes for radio light use the bottom portion of the paraboloid. Radio dishes may not look like traditional mirrors, but they’re reflective to radio waves.
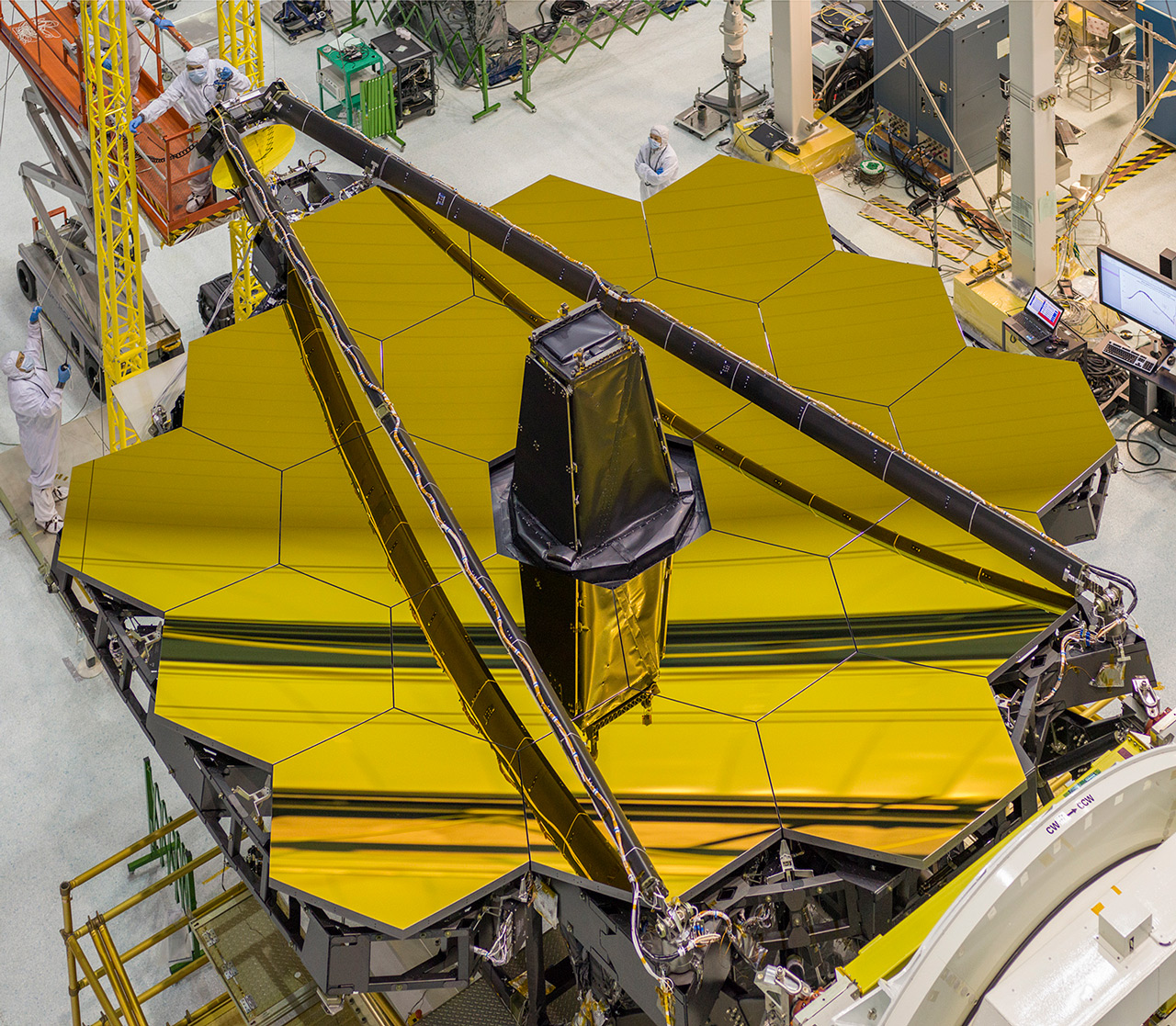
For example, the golden honeycomb of the James Webb Space Telescope is made of individual panels that combine to make one enormous mirror designed to collect infrared light from some of the oldest stars and most distant objects in the universe. The Hubble Space Telescope also uses this cup shape to collect ultraviolet, visible, and infrared light.
Telescope mirrors are coated with different materials depending on the color of the light they need to reflect. The Webb mirror, for example, is coated with a thin layer of gold so that it can reflect infrared light.
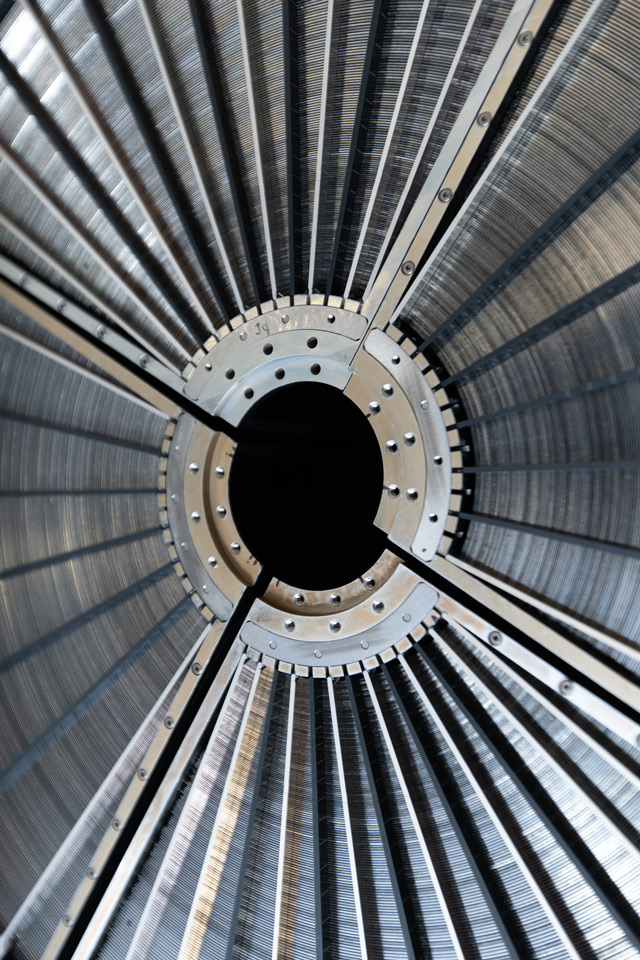
X-ray mirrors use the slightly angled side of the paraboloid. X-rays can simply pass through the atoms that make up most telescope mirrors. To detect them, engineers turn the mirrors on their sides so the X-rays can skip off the surface. This is called a grazing incidence mirror. Since there’s a lot of empty space in the middle of a single paraboloid, X-ray mirrors incorporate multiple mirrors as nested onion-like shells. Engineers at NASA’s Goddard and Marshall Space Flight Centers have designed mirrors like these for missions like the X-ray Imaging and Spectroscopy Mission (XRISM) and the Imaging X-ray Polarimetry Explorer (IXPE).
Finding gamma rays
Gamma rays are the universe’s most energetic form of light. They go straight through optics used for other wavelengths, making them impossible to reflect or refract. Instead, astrophysicists use special detectors to observe gamma rays and to figure out where they come from in the sky.
For example, the Fermi Gamma-ray Space Telescope's Large Area Telescope (LAT) detects particles produced in a process known as pair production. When a gamma ray hits a layer of tungsten in one of LAT’s tracking towers, it creates an electron and its antimatter counterpart, a positron. (This process epitomizes the famous E=MC^2 equation.) Several layers of high-precision silicon detectors track the particles as they move through the instrument. The direction of the incoming gamma ray is determined by projecting the particle paths backward. The particles travel through the trackers until they reach a separate detector called a calorimeter, which absorbs them and measures their energies.