Ocean Physics at NASA
NASA's Ocean Physics program directs multiple competitively-selected NASA Science Teams that study the physics of the Ocean.
About Ocean Physics
The ocean plays a fundamental role in the Earth’s system. It shapes our planet’s climate and weather by absorbing, storing, and transporting large quantities of heat, water, moisture, and carbon dioxide. NASA’s Ocean Physics program enables research that advances our understanding of the ocean’s role in climate. Our scientists utilize NASA satellite and sub-orbital data, climate models and data science to study ocean dynamics, sea level rise, hydrological cycle, and ocean interactions within the Earth system.
Apply to one of the current NASA research opportunities to join us as a NASA scientist.News
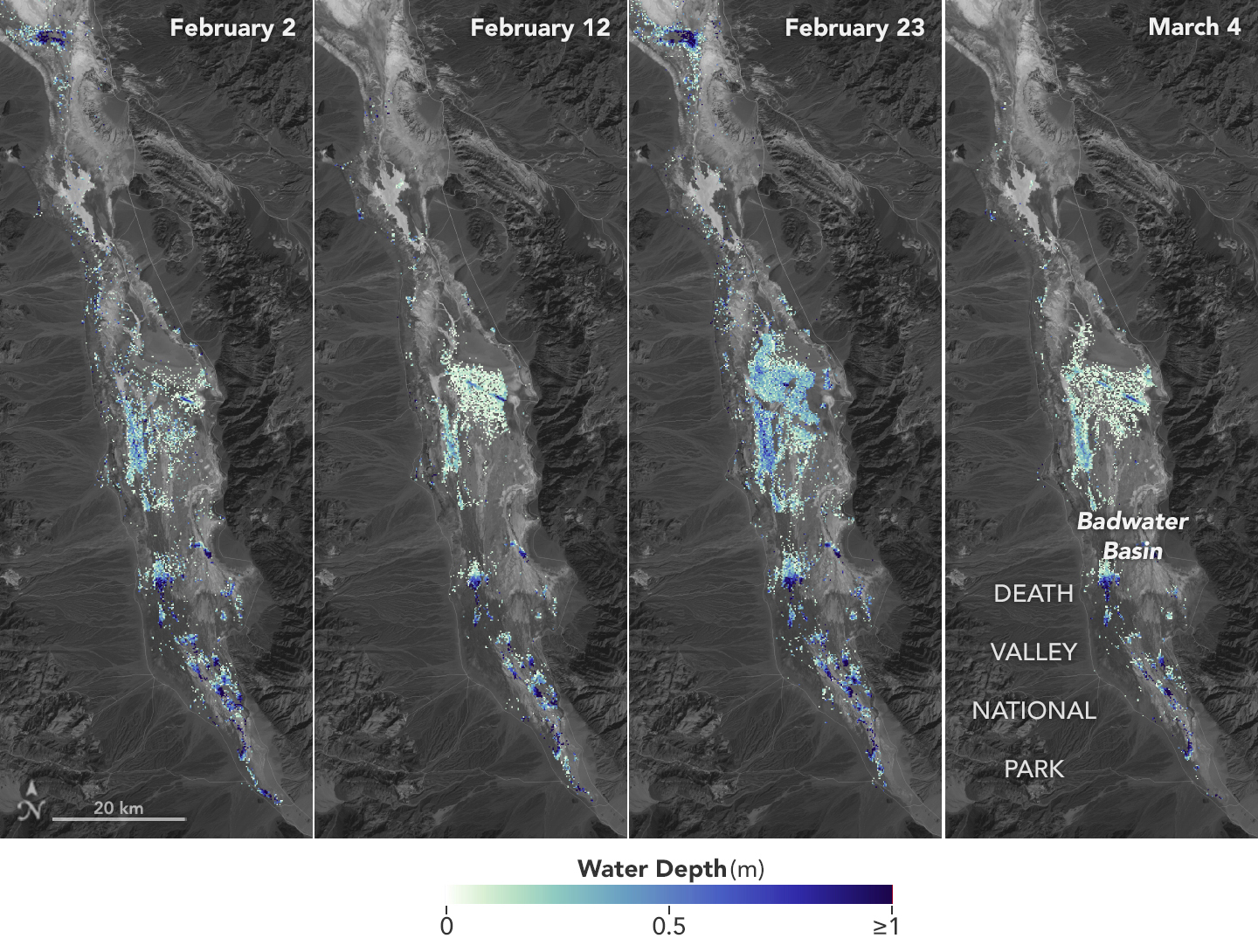
SWOT measures Death Valley’s temporary lake
Changes in ephemeral lake in Death Valley is measured by SWOT.
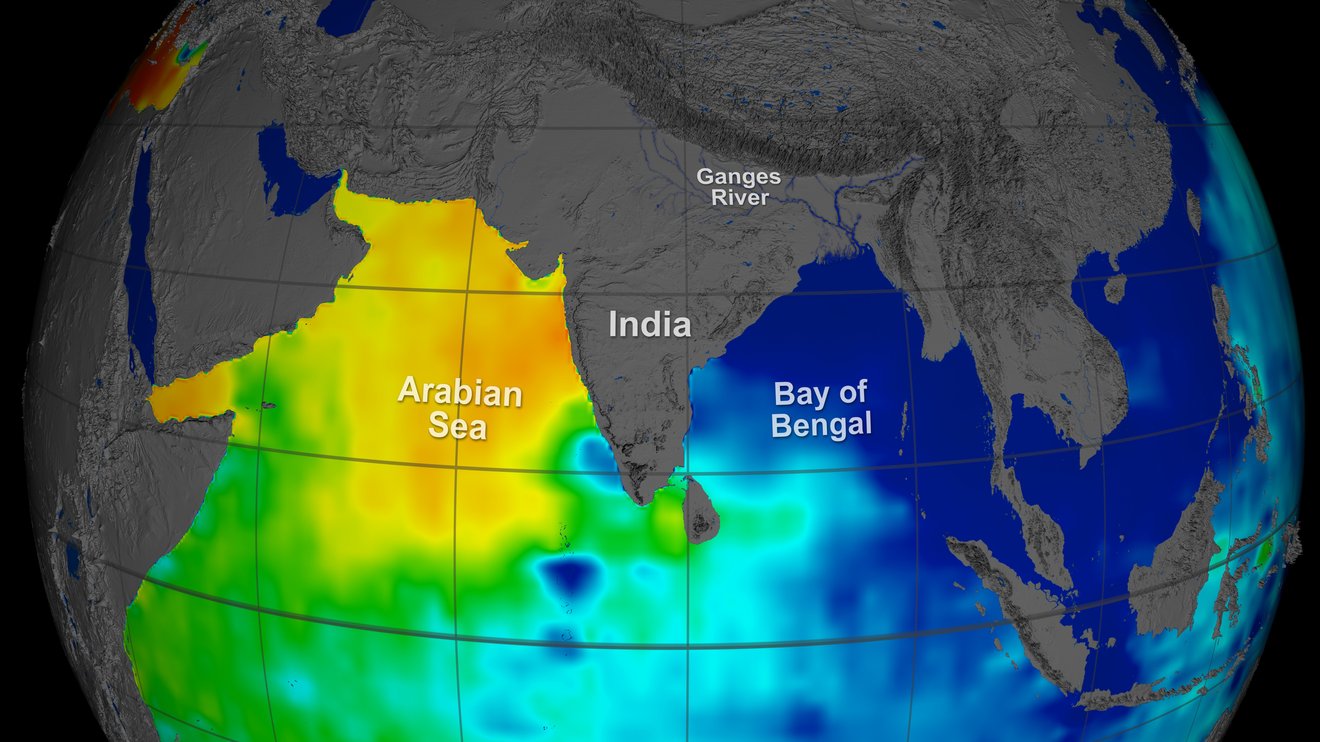
How NASA Spotted El Niño Changing the Saltiness of Coastal Waters
New findings have revealed a coastal realm highly sensitive to changes in runoff and rainfall on land.
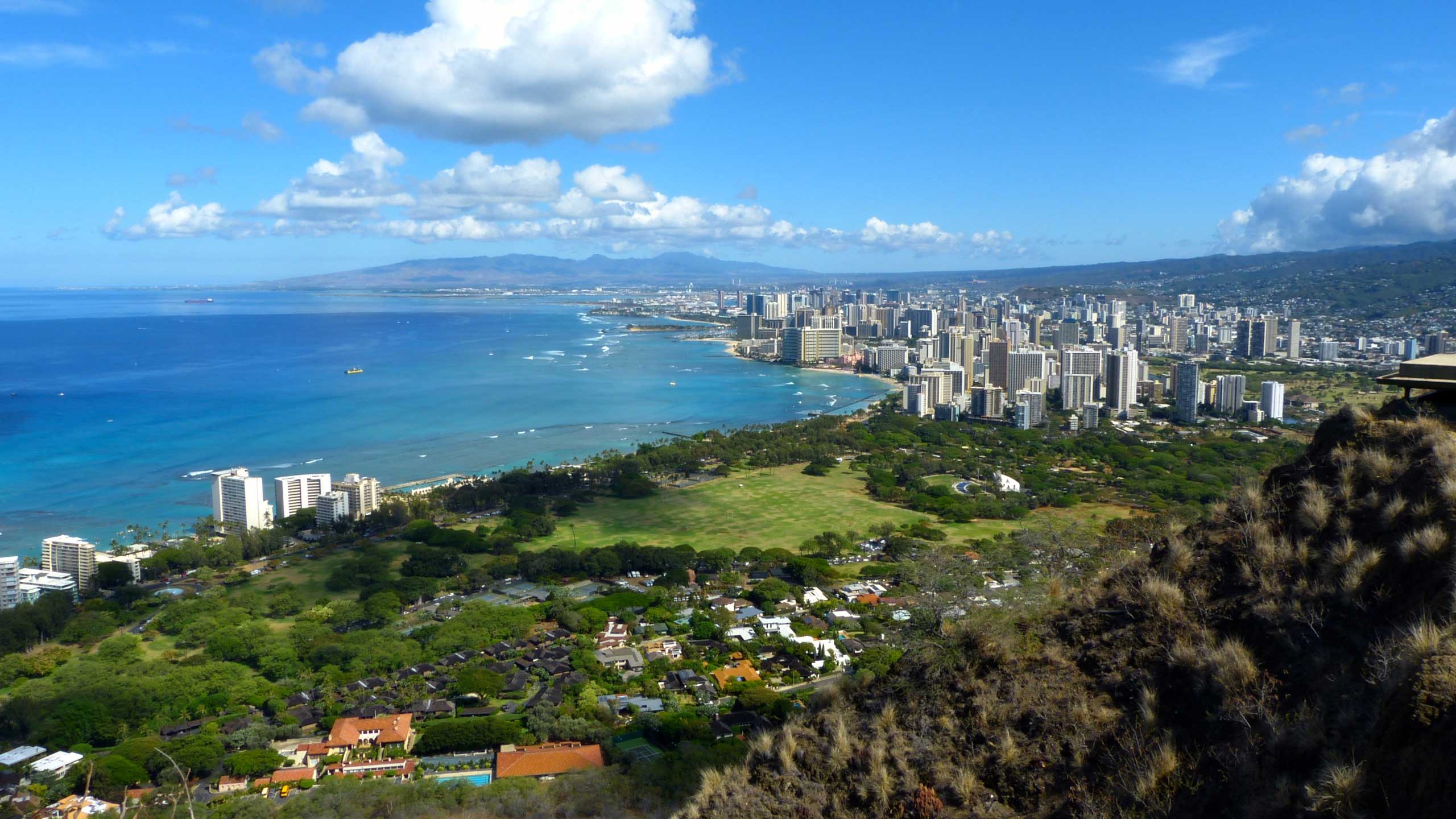
NASA Analysis Sees Spike in 2023 Global Sea Level Due to El Niño
A long-term sea level dataset shows ocean surface heights continuing to rise at faster and faster rates over decades of observations.
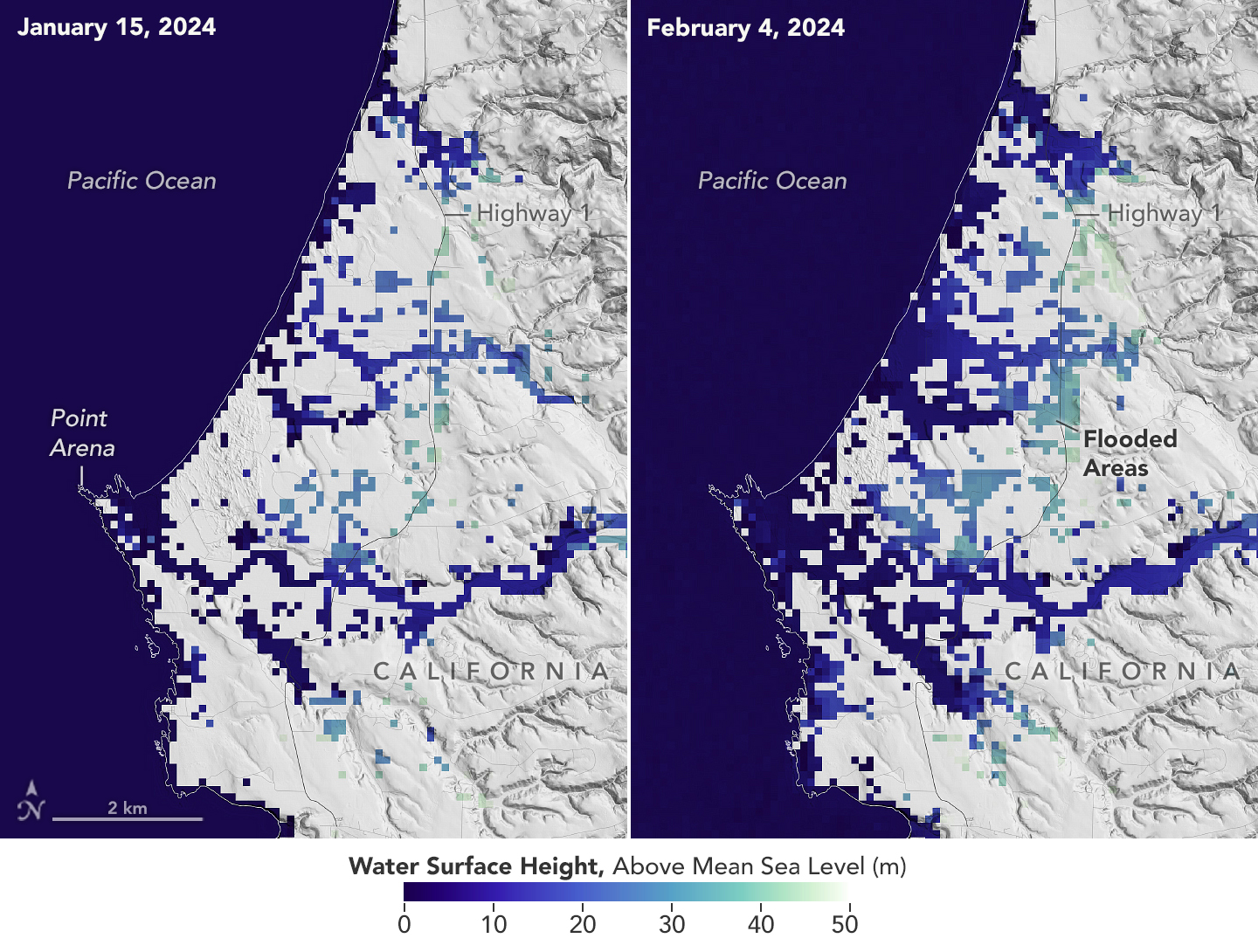
SWOT Satellite Catches Coastal Flooding During California Storms
SWOT mission provides a new view of water on land, at the coast, and in the ocean.
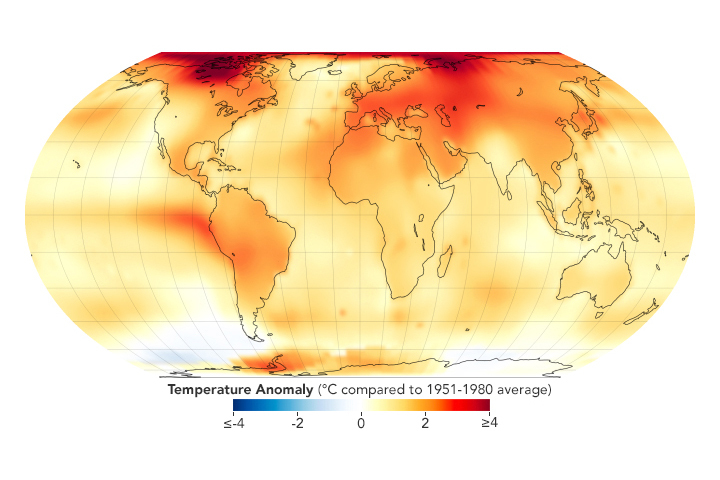
NASA Analysis Confirms 2023 as Warmest Year on Record
Earth’s average surface temperature in 2023 was the warmest on record, according to an analysis by NASA.
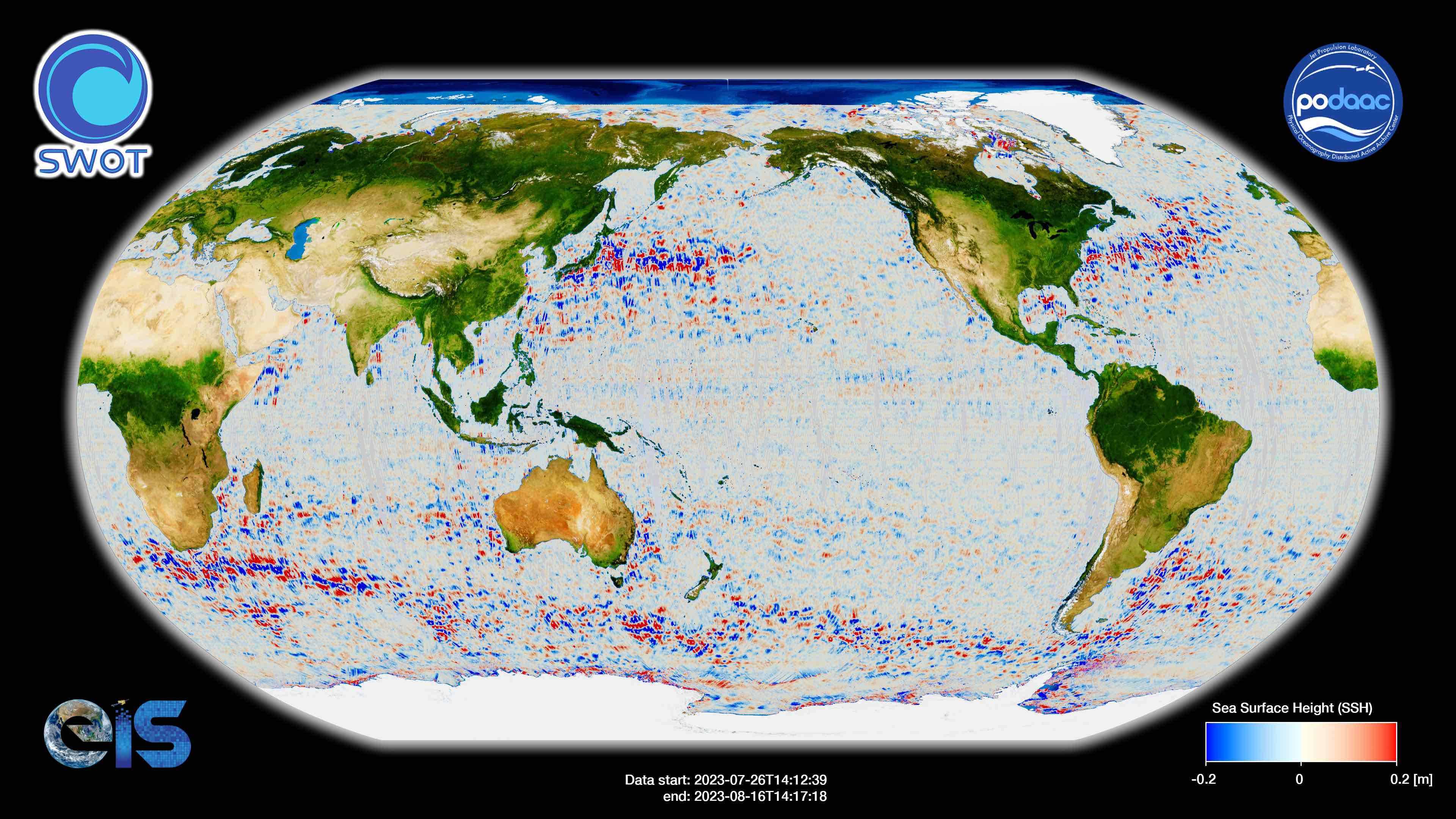
SWOT Data: First Public Release
Some of the first data products from the Ka-Band Radar Interferometer (KaRIn) on the SWOT satellite are now publicly available.
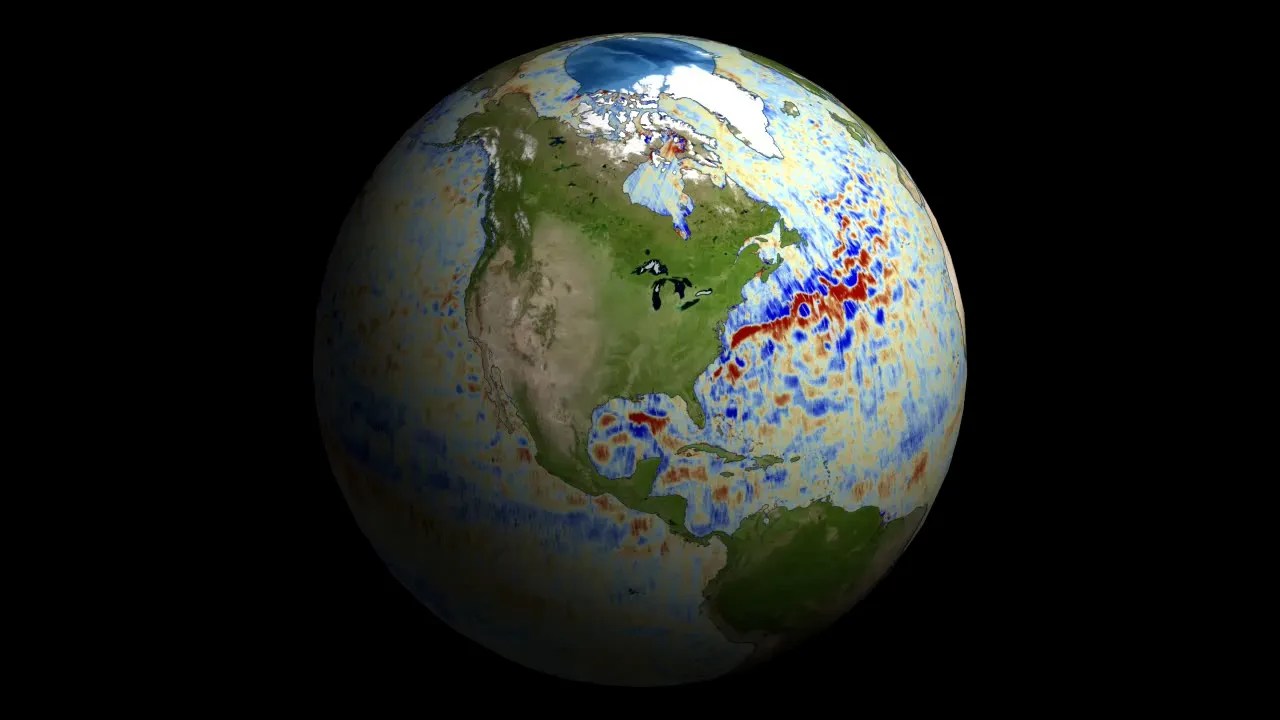
SWOT’s Unprecedented View of Global Sea Levels
Data on sea surface heights around the world from the international SWOT (Surface Water and Ocean Topography) mission yields a mesmerizing view of the planet’s ocean.
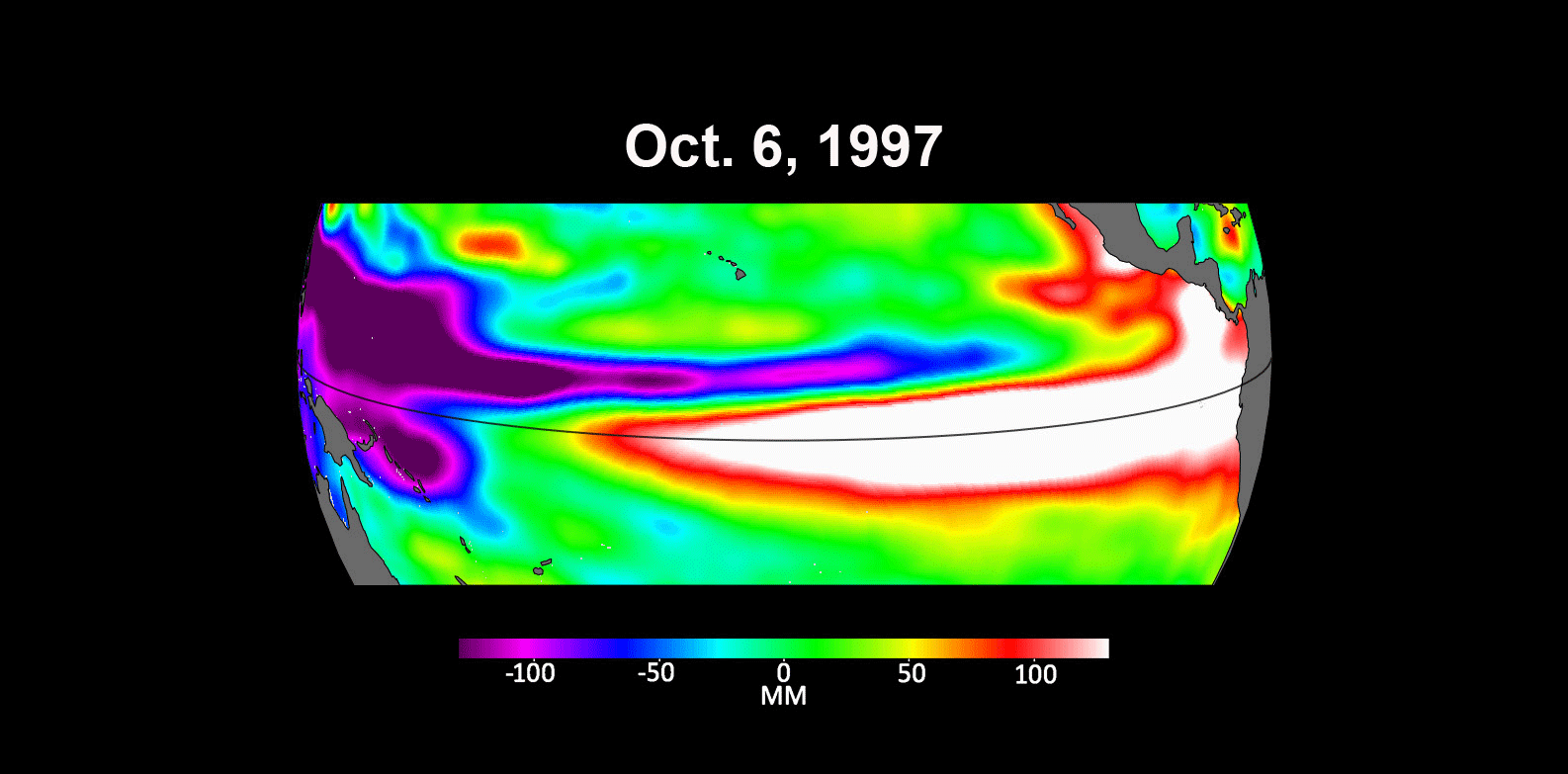
Sentinel-6MF Monitors How El Niño Is Shaping Up
Sentinel-6 Michael Freilich is the latest satellite that researchers are using to compare this year’s El Niño with those of the past.
Science and Research
NASA’s Ocean Physics program directs multiple competitively-selected NASA’s Science Teams that study the physics of the oceans. Below are details about each science team.
Missions
The Ocean Physics program currently provides scientific leadership for the following operational missions. Past and future missions (to be) lead by the Ocean Physics program are also highlighted separately.
Operational
Currently taking measurements
Future
Upcoming missions
Non-Operational
Past missions providing data for current research
Access the Data
The Physical Oceanography Distributed Active Archive Center (PO.DAAC) preserves NASA's ocean and climate open data and makes these universally accessible and meaningful.
Partnerships
The Ocean Physics program actively engages in the following national and international partnerships that work together to sustain and enhance the Earth observing system.
![]() NASA-ESA Earth Science Joint Program Planning Group NASA-ESA Satellite and In situ Salinity Working Group |